Introduction:
Secondary metabolites represent phenolic compounds as are main class. Among these compounds, flavonoids are the most common and widely distributed class of plant phenolics (Brodowska, 2017); about 10,000 flavonoids have been reported (Santos, Maia, Ferriani, & Teixeira, 2017). Flavonoids are classified into ten chemical groups, and different flavonoids (over 5000) have been discovered to date (Khan, Al-Daghri, Al-Ajlan, & Alokail, 2014).
According to the molecular structure of flavonoids, they are classified into six different classes: flavonols, flavones, flavanols, flavonones, isoflavonones and anthocyanins (Ross & Kasum, 2002). Flavonoids availability in plants plays many physiological roles, as they exhibit anti-oxidant, anti-cancer, anti-bacterial, and anti-ageing properties (Liu et al., 2012). Many isolated flavonoids exhibit different pharmacological activities including anti-cancer, (Zhang et al., 2017) anti-inflammatory, (Saini, Gahlawat, & Lather, 2017), anti-allergic, (Tanaka et al., 2003), anti-viral, (Sanchez, Gómez-Garibay, Taboada, & Ruiz, 2000; Sánchez-Roque et al., 2017) and anti-oxidant activities (Braca, Sortino, Politi, Morelli, & Mendez, 2002). Some common uses of pure flavonoids are to treat cardiovascular and gastrointestinal disorders. Flavones and flavanones found in different fruits and vegetables exhibited potent biological activity, including anti-cancer, anti-diabetic, anti-inflammatory, anti-hypertension, relief from obesity, and health effects on cardiovascular disease (Pandey, Gurung, & Sohng, 2015).
In this study, we use the concept of natural product-based drug discovery since nitrogen-containing heterocyclic compounds play a vital role in the medicinal field (Liaqat et al., 2017). The nitrogen moiety was introduced in the plant-based compounds to enhance its biological activity or search for other medicinally essential compounds. For this purpose, we used the mannich reaction method to form a carbon-nitrogen (C-N) bond. Since mannich reaction obtains importance in the chemical and pharmaceutical field (Armstrong, Combs, Tempest, Brown, & Keating, 1996). Mannich reaction is the three-component reaction of amine (primary or secondary), aldehyde and carbonyl compounds that form mannich bases (Haji, 2016), which shown different biological activities such as anti-cancer (Lopes et al., 2004), anti-inflammatory (Sujith, Rao, Shetty, & Kalluraya, 2009), anti-bacterial (Kantlam, Murthy, & Reddy, 2015), anti-fungal (Ashok, Holla, & Poojary, 2007), anti-tubercular (Joshi, Khosla, & Tiwari, 2004), anti-malarial (Lopes et al., 2004) and anti-analgesic drug (Malinka et al., 2005).
Neoplasm, a group of more than 270 diseases affecting all age groups and almost all body tissues, organs, and systems, is a multi-factorial, multi-step, multistage accruing illness that initiates after multiple genetic and epigenetic modifications in the normal nuclear makeup of cells usually characterized by unchecked, unusual and uncontrolled proliferation (Hanahan & Weinberg, 2011; Hassanpour & Dehghani, 2017; Heng et al., 2010). Tumorigenesis is a multifaceted and multistage process that involves the unusual multiplication of a group of cells in a specific body part after a significant driver mutation. The cellular DNA repair mechanism fails to fix such transformations. That sort of cells increases their population and pass on mutated genetic material to lineage (Kim, 2015). Further mutations in these cells lead to heterogeneity, a feature of neoplasm. Such cells produce larger populations, start affecting nearby cells, enlarge their size, develop new blood vessels and consequently invade other body parts or systems, a characteristic of malignancy known as metastasis. Metastatic malignancy is life-threatening and requires rigorous treatment, intensive care and critical monitoring of untoward effects (Kim, 2015).
Surgical removal, radiation therapy, immunotherapy, hormonal starvation therapy, targeted therapy, stem cell grafting, and chemotherapy are the treatment options for cancer (Miller et al., 2016). Chemotherapy is the most widely adopted treatment strategy for cancer, either alone or with other therapies. Both natural and synthetic compounds are currently in practice to combat cancer, but conventional chemotherapeutic approaches cannot control the incidence of most cancer types. Introducing novel compounds with improved pharmacological actions is a need of the hour. Synthetic chemistry has an edge to synthesize natural compounds on a larger scale economically, thus helping in the continuous supply of life-saving medicines (Dua, Shrivastava, Sonwane, & Srivastava, 2011; Maccoss & Baillie, 2004). Compounds of natural origin have always been a mainstay of lead compounds (Gordaliza, 2007). Organic chemical synthesis provides an opportunity to manufacture and modify natural leads by structural changes and substitutions to analyze such compounds extensively in search of more specific, effective and safer chemotherapeutics (Blagosklonny, 2005; Shang & Tan, 2005; Yeh & Lim, 2007). In the current study, derivatives of flavons and flavanones were synthesized by a Mannich reaction. In flavones, the reaction occurs at the C-8 position. This is because of the π–conjugation of the hydroxyl group at C-5 and C-7 in ring A (Liu et al., 2012). While in flavanone, the reaction happens at C-8 and C-4 positions because of the enolizable proton at that position. The MTT cell viability assay was used to test the anticancer potential of these flavones, flavanones, and derivatives on HeLa and MCF-7 cancer cells. The apoptotic impact was validated using propidium iodide (PI) and DAPI staining. It was also tested for cell cycle, reactive oxygen species, lactate dehydrogenase, mitochondrial membrane potential, and caspase-9 and -3 activity.
Materials and Methods
Experimental material
Naringenin and Chrysin were isolated from Callistephus chinensis and Flavanone from Ocimum basilicum. All other chemicals and solvents that were used in this research were purchased from Sigma Aldrich. NMR was recorded on Brucker. 1HNMR and 13CNMR spectrometers were operated at 400 and 150 MHz. The value of chemical shift and coupling constants were expressed in ppm and Hz. Mass spectra were recorded using EI and ESI-MS spectrometer.
Experimental material and procedure for anticancer activity was followed by previous procedure (Lin et al., 2013; Rastogi, Singh, Hader, & Sinha, 2010; S et al., 2021; Scifo et al., 2004; Uddin et al., 2020)
Synthesis ofMannich base derivatives
Synthesis of Compound 2
In a two necked 250ml round bottom flask, Chrysin that is mentioned as compound 1 (100 mg, 0.39mmole) were dissolved in DMF (1ml), and Methanol (15ml) was added as a solvent, all the reagent kept on oil bath with constant stirring then dicyclohexylamine (600µl, 0.58mmole), formaldehyde solution (200µl, 1mmole) were added dropwise to the reaction mixture. The reaction was allowed to get the homogenized mixture. After 2 hours, yellow precipitates were formed, and precipitates were filtered through filter paper. The pure yellow color residue was collected with a 59.7% yield.
Synthesis of Compound 4
Flavanone (100mg, 0.45mmole) was dissolved in anhydrous DMF (1ml) and Methanol (15ml) in a two necked round bottom flask and immersed in an oil bath with constant stirring for 12 hours and then the solution of Dicyclohexylamine (700µl, 1.3mmole) and formaldehyde solution (50µl, 1.7 mmole) were added in a reaction mixture and kept in an oil bath to get homogenized mixtures and the overall reaction was monitored through TLC. After 12 hours, the reaction was halted and filtered through filter paper, followed by the collection and drying of the supernatant under decreased pressure. Purification of the product using PTLC (2 percent Methanol: 98 percent DCM) yielded compound 4 in 44 percent yield.
Synthesis of Compound 6 and 7
Naringenin (100mg, 0.37mmole), DMF (1ml) and Methanol (15ml) were taken in two necked round bottom flasks. The reaction was kept on an oil bath with continuous stirring for 2 hours, then Dicyclohexylamine (110µl, 0.5mmole) and formaldehyde solution (50µl, 1mmole) was added to the reaction mixture. After the completion of the reaction, the reaction mixture was filtered through filter paper, and the supernatant was collected. It was purified using column chromatography after solvents were evaporated at decreased pressure (ethyl-acetate and hexane), and we got the two compounds (6 and 7) with a 23% yield.
Result and Discussion
Synthesis of Mannich Bases
Flavones and flavanones are considered antioxidant compounds, and the Mannich reaction synthesized their derivatives. For the structure-activity relationship, we checked all the isolated and their derivatives for anticancer activity. The compounds (mentioned 1, 3, and 5) were isolated from a plant source and used to synthesise compounds (mentioned 2, 4, 6 and 7) using dicyclohexylamine, formaldehyde, dimethylformamide, and methanol as solvent Scheme 1 and 2 represents their synthesis.
All the isolated and synthesized compounds were characterized by using UV, EI-MS, 1HNMR, 13CNMR techniques. The purity of compounds was determined by TLC. All synthesized mannich bases were purified by normal phase column chromatography except compound 2 purified by the precipitation method. The 1HNMR data for the isolated compounds are presented in Table 1. The characteristic peak of the newly synthesized compounds is represented in Table 2 and in Appendix A.
Table 1
1HNMR values of natural product in DMSO-d6 at 500 MHz.
Table 2
1H and 13CNMR values of mannich bases in DMSO-d6 at 500 and 150 MHz.
Anticancer activity
All the isolated and derivatives cell viability was explored on HeLa and MCF-7 (cancer cell lines) and BHK-21 (normal cell line). Compounds 1,6 and 7 showed more than 50% inhibition against HeLa, MCF-7 and BHK-21 cells, as shown in Table 3. Compound 7 was selected to investigate its mechanism of action through various assays, as shown in Figure 4; Figure 3.
Figure 1
Propidium iodide staining with flow cytometry. G0/G1,S and G2/M stages in HeLa cells treated with cisplatin, untreated and compound 7.
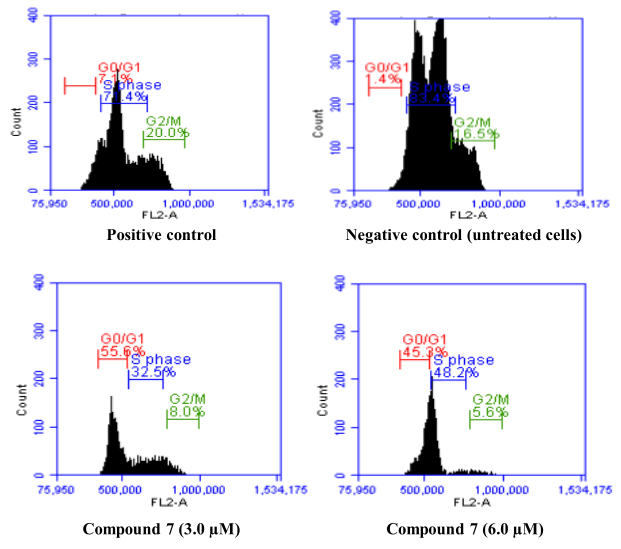
Figure 2
Induction of nuclear morphology after treatment with compound 7 and labelling with DAPI (upper image) and PI (lower image) in HeLa cells.
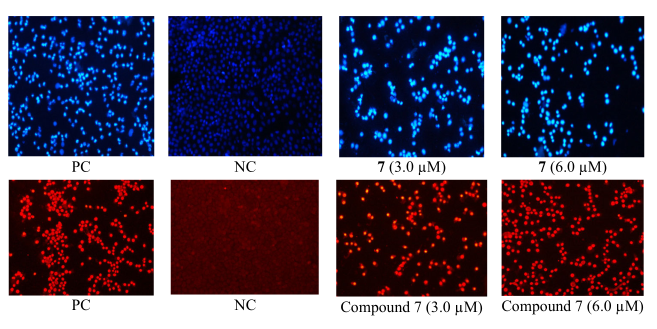
Fluorescence DCF oxidation in HeLa cells induced by 2 × IC50 values of compound 7, cisplatin, and untreated cells following exposure to 2',7'-dichlorofluorescein diacetate. The apoptotic impact of compound 7 was demonstrated in Figure 5.
Figure 3
HeLa cells treated with compound 7, cisplatin, or 2',7'-dichlorofluorescin diacetate show oxidised DCF fluorescence. Photographed in HeLa cells with a fluorescent microscope.
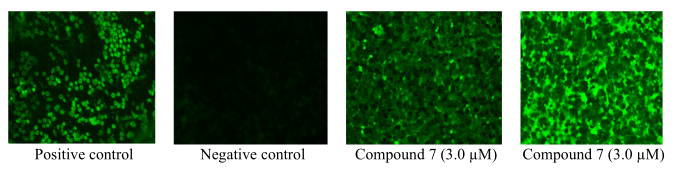
Caspase 8, 9 initiates the executioner caspase 3, 6 and 7 that has the prompt part in activating apoptosis. Compound 7 demonstrated a reduction in the mitochondrial membrane potential in HeLa cells, indicating that it may have triggered apoptosis via the intrinsic mechanism. (Figure 8; Figure 7; Figure 6).
Figure 6
Compound 7 has an effect on the mitochondrial membrane potential in HeLa cells. Untreated cells retained a normal ΔΨm, whereas treated cells exhibit a compromised ΔΨm.
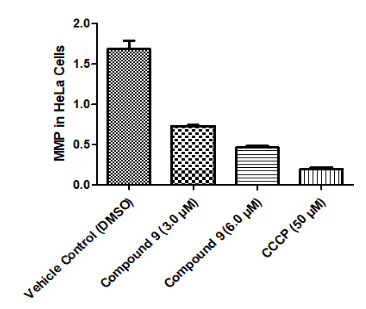
Using propidium iodide PI) and DAPI staining as fluorescence microscopic imaging was confirmed the apoptotic effect of potent compound 7. Further, it was evaluated by cell cycle analysis through flow-cytometry, reactive oxygen species and lactate dehydrogenase production. The caspase-9 and -3 activity were estimated by mitochondrial membrane potential (S et al., 2021; Uddin et al., 2020).
Table 3
Inhibitory concentrations of pure compounds and cisplatin in HeLa, MCF-7, and BHK-21 cells.
Conclusion
Flavones and flavanones are two primary classes of Flavonoids, considered anti-oxidant compounds. In order to enhance their biological activity and checked structure-activity relationships, their Mannich bases were synthesized by Mannich reaction and evaluated for cancer activity. Three out of seven compounds exhibited more than 50% inhibition of cancer cell lines, and these compounds were evaluated for cytotoxic potential towards normal cell lines. MTT assay results exhibited that the cytotoxic profile of compound 7 against cancer cells is very appealing. The compound has proved its capability to produce toxic effects in cancerous cells by targeting mitochondria, inducing ROS, releasing lactate dehydrogenase, activating caspases, and causing cell cycle arrest in cancer cells. Therefore, it may be suggested that our compound has the potential in inducing apoptosis.