INTRODUCTION
Malignant melanoma is the most lethal form of skin cancer and is the fifth most common cancer in women and in men, and the global incidence continues to increase (ACS, 2022; Siegal, Miller, Fuchs, & Jemal, 2022). Staphylococcus, a major bacterial human pathogen that causes a wide variety of dangerous clinical manifestations, and Streptococcus, a host of the mucosal surfaces of humans, are a Gram-positive bacteria responsible for the pathogenesis of a variety of diseases such as wound infections, mastitis, toxic shock syndrome, scarlet fever, cystic fibrosis, nosocomial and community-acquired infections, cancer, hepatobiliary disease, colorectal lesions, periodontitis, tonsillitis, endocarditis, pharyngitis, meningitis, glomerulonephritis and indwelling device-associated infections (Cebeci & Kırmusaoğlu, 2020; Taylor & Unakal, 2019).
Much is investigated to understand the bioactive activity of plants against various pathologies. The WHO Traditional Medicine Strategy 2014-2023 aims to develop proactive practices and implement action plans that will strengthen the role that traditional and complementary medicine plays in keeping populations healthy (WHO, 2013). Plants are an important source of natural compounds used for medicine, with considerable interest and special attention for their wide variety of bioactive metabolites. Several phytochemicals of different species have antitumor (Enaru et al., 2021; George, Chandran, & Abrahamse, 2021; Hu et al., 2022; Khan et al., 2020; Nascimento, Reguengo, Machado, & Junior, 2022; Patra et al., 2021) and antimicrobial activity (Christenhusz & Byng, 2016; Fernández et al., 2020; Marco, Estrada, & Pungitore, 2019; Vakayil et al., 2021).
Annonaceae include more than 2,500 species distributed in more than 100 genera (Christenhusz & Byng, 2016; Handayani & Yuzammi, 2021). Many studies point to the antitumor potential of several Annonaceae species on different cancer cell lines (Al-Nemari et al., 2022; Behl, Inbanathan, Sundaram, & Hussain, 2022; Swapna, Rao, Kotha, Sagar, & Setty, 2022). A few scientifically studied species of the Rollinia (Annonaceae) genera exhibit phytochemicals with antimicrobial potential including Staphylococcus spp. (Fernández et al., 2020; Marco et al., 2019) and anticancer potential (Aumeeruddy & Mahomoodally, 2021; Muhartono, Rachmawati, Jaya, & Putra, 2018; Muhartono, Subeki, & Hanriko, 2019; Muhartono, Sukohar, Sutyarso, & Kanedi, 2016; Neske, Hidalgo, Cabedo, & Cortes, 2020; Pham, Le, Le, Vo, & Ho, 2020; Shi, Macdougal, & Mclaughlint, 1997) including melanoma (Ceramella et al., 2021; Cunha et al., 2009). Leaf extracts of Annona crassiflora that had tannins (Ribeiro et al., 2018; Sieniawska & Baj, 2017; Smeriglio, Barreca, Bellocco, & Trombetta, 2017) and xanthone derivatives removed, no inhibitory effects on bacterial growth were observed (Ribeiro et al., 2018). Alkaloids, phytochemicals present in many annonaceae (Dev & Joseph, 2021; Justino et al., 2021; Perrone, Yousefi, Salami, Papini, & Martinelli, 2022; Verma, 2020), also exert antibacterial action including Staphylococcus (Marco et al., 2019; Perrone et al., 2022).
This is the first study of the antitumoral, antioxidant and antibacterial bioactivity of R. dolabripetala leaf extracts (RDLE). The present study investigates the antioxidant, anti-melanoma, anti-staphylococcal and anti-streptococcal potential of hydroalcoholic extracts from the leaves of R. dolabripetala, the polyphenolic compounds 1.7-dihydroxyxanthone and xanthone, as well as the chemotherapeutic 5-Fluorouracil.
MATERIALS AND METHODS
Botanical material
R. dolabripetala (Raddi) R.E.Fr. (Annonaceae) leaves were collected (October and November) in a rural area of the Subperenifolia Atlantic Rainforest, 649 meters above sea level, in the municipality of Viçosa, Minas Gerais, in the Mata Mineira Zone (20º 45' 14” S and 42º 52' 54” W), with a tropical altitude climate predominance. Voucher specimens were deposited in the VIC Herbarium of the Federal University of Viçosa, Minas Gerais, under the registration code Rollinia dolabripetala (Raddi) R.E.Fr. (VIC: 35.760).
Preparation of the hydroalcoholic extract
R. dolabripetala leaves were washed in 1% sodium hypochlorite and subsequently kept in an oven at 45 ºC for drying. The procedure was concluded when the mass variation did not exceed 0.005 g in a 12 hour period, where the material was then subjected to electric grinding. The hydroalcoholic extracts of the leaves were prepared using the maceration method (Simões et al., 2002) and then subject to an alcoholization in a 1:1 ratio (water and ethyl alcohol) for 72 hours. After that time, the supernatant was filtered and separated. A new alcoholic solution was added to the saturated solution and repeated until the extraction of the organic matter extract was complete. The filtrate had all the solvent extracted by the rotary vaporization method (50 ºC) and the aqueous solution was frozen at -20 ºC for 24 hours and at -80 ºC for an additional 24 hours before being subjected to lyophilization for 8 hours. The lyophilized extract yield was calculated using the following equation: (%) yield = (weight of lyophilized extract / weight of fresh material) x 100.
Preliminary phytochemical screening
The secondary metabolites like phenols and tannins, leucoanthocyanidins, anthocyanin, flavonols, flavanones, flavanonols, xanthones, saponins and anthraquinones were evaluated (Harborne, 1998). The tests were carried out in triplicates.
Determination of antioxidant capacity by the DPPH method
The percentage of 2.2-diphenyl-1-picrilhhydryl (DPPH) radicals captured after a determined amount of time was analyzed (Pukalskas et al., 2002). The DPPH solution was prepared from 0.1 mmoL/L of DPPH• in 80% ethanol: water (v/v). Then, 50 mg of each extract was diluted in an 80% water: alcohol solution (v/v) and, from this stock solution, 100 μL were aliquoted and diluted in the proportion of 1:120. In a test tube, an aliquot of 0.5 mL of the fraction of the samples was added (properly diluted so that partial reduction of DPPH occurs) and 3.5 mL of the ethanolic solution of DPPH at room temperature (25 ± 1 ºC). The entire experiment was carried out in an environment devoid of light and the stabilization of the absorbance value (517 nm) occurred when the result varied less than 0.005 (UV-1601 PC Shimadzu®). The result was expressed as a percentage of DPPH radical molecules captured, at a given dilution, by the equation: (%) sequestering activity = 1 – [•DPPHf / •DPPH0] x 100, where (•DPPH)0 and •DPPHf absorbance in the start and end of the reaction in a pure DPPH sample.
Determination of antioxidant capacity by the lipid peroxidation method
The oxidative degradation of lipids was based on peroxidation in liposomes by the malondialdehyde test (MDA C3H4O2) (Benzie, 1996; Janero, 1990). Test tubes containing 0.5 mL of the liposome solution (10 mM) received 100 μL of each experimental extract (0.2 mg/mL) dissolved in dimethylsulfoxide (DMSO), 100 μL of the positive control di-tert-butylmethylphenol (BHT) and 100 μL DMSO (0.5% ± 0.06) as a negative control. Lipid peroxidation was induced by adding 125 μL of FeCl3 ferric chloride (0.2 mM) and 1 mL of ascorbic acid (10 mM) to all tubes. Then, all tubes were incubated under shaking at 40 ºC for 60 min. After that time, the reaction was stopped by adding 1 mL of thiobarbituric acid (TBA) at a concentration of 0.375% dissolved in 15% trichloracetic acid (TCA-HCl) in HCl (0.25 mol/L). The tubes were then heated in a thermostatic bath at 80 ºC for 15 min and, later, centrifuged at 3,000xg for 15 min at 15 ºC. The reading of the absorbance of all samples (532 nm) had the “white” level containing water, ferric chloride, ascorbic acid and TBA as the leveling standard. The peroxidation index in TBA was expressed in terms of the percentage of peroxidation inhibition of each extract (Buege & Aust, 1978). The calculation of the results was obtained by the average of the triplicates of all experimental tests. Using water as a control, the percentage of inhibition in lipid peroxidative activity was calculated by the absorbance (abs) according to the equation: (%) inhibition = [(final average abs of the control – average abs of the sample) / average abs of the control] x 100.
Cell viability and cytotoxicity test
The murine melanoma cell line B16 variant F10 (B16F10 - ATCC® CRL-6475) was maintained in a DMEM culture medium (Gibco®) supplemented with L-glutamine (2 mM), penicillin (100 IU/mL), streptomycin sulfate (100 μg/mL), NaHCO3, (2.2 g/L), 10% fetal bovine serum (Cultilab®), at pH 7.2, incubated at 3 x 103 cells/well in 96-well plates (TPP®), at 37 ºC in a humidified atmosphere containing 5% CO2 (Thermo Scientific HEPA Class 100®). The viability of B16F10 melanomic cells was evaluated using the trypan blue technique (3 x 103 cells/mL) in 96-well plates (TPP®). After 24 hours, the culture medium was replaced by another medium containing increasing concentrations of secondary phytometabolites 1.7-dihydroxixanthone (Figure 1a), xanthone (Extrasynthese®) (Figure 1b), the chemotherapeutic 5-Fluorouracil (Pharmanostra®) (Figure 1c), and extracts from R. dolabripetala leaves, all initially diluted in DMSO (0.5% ± 0.06). After 72 hours of treatment, the culture medium was removed and the plate was washed twice with PBS (100 μL/well) for testing with MTT (0.5 mg/mL) at 550 nm. In this first stage, the inhibitory concentration of 50% viability of melanoma cells was calculated, with the tests always performed in triplicates, in the periods of 24, 48 and 72 hours. The analysis of the cytotoxic activity of the extracts was based on the MTT colorimetric evaluation [3-(4.5-dimethyl-2-thiazol) 2.5-diphenyl-2-H-tetrazolium bromide] (Mosmann, 1983). The ability of the tested compounds to inhibit 50% of cell growth (GI50) was calculated using the result of the MTT test (Sigma Aldrich®) using the GraphPad Prim® 5.0 program.
Figure 1
Structure of the (a) xanthone (Molecular formula: C13H8O2; Mol weight: 196.2 g/mol), (b) 1.7-dihydroxyxanthone (Molecular formula: C13H8O4; Mol weight 228.2 g/mol), and (c) 5-Fluorouracil (Molecular formula: C4H3FN2O2; Mol weight: 130.08 g/mol) compounds (Wiley & Sons, 2022).
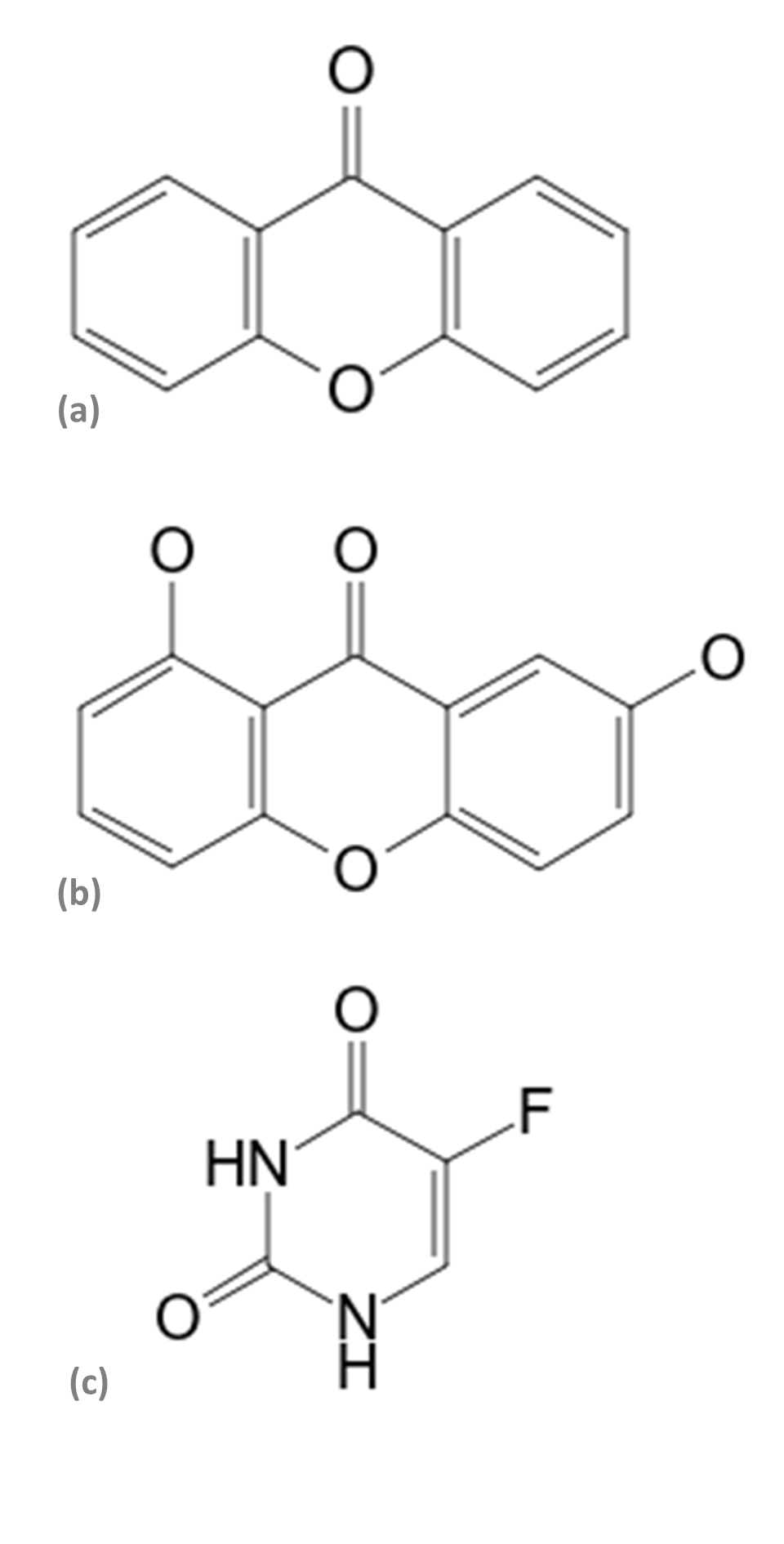
Evaluation of antimicrobial activity
Gram-positive strains Staphylococcus aureus subsp. aureus Rosenbach ATCC® 29213 (SA13), S. aureus 4075 (SA75) and Streptococcus bovis 01 (SB01) were used for experimental antibacterial assays. The SA13 strain was supplied by the Animal Virology Laboratory of the Veterinary Department of the Federal University of Viçosa; the SA75 and SB01 were isolated from bovine mastitis at the Brazilian Dairy Cattle Agricultural Research Corporation Embrapa, Coronel Pacheco unit, Minas Gerais. The indicator microorganism was cultured in Brain Heart Infusion broth (Difco™), for 24 hours at 37 oC, later transferred to test tubes containing 5 mL of sterile saline solution. The suspensions were standardized using the McFarland scale (0.05 mL of 1% barium chloride + 9.95 mL of 1% sulfuric acid). Thus, an inoculum containing approximately 108 cells/mL ('concentrated suspension') was obtained, added to the Müeller-Hinton medium (Difco™) in the proportion of 2%. These suspensions were kept in a water bath (50 ºC) until the moment of use, not exceeding 60 min. For the qualitative test, the well diffusion method was used. Bacterial strains were exposed to different concentrations of RDLE ranging from 0.1 to 5.0 mg/mL. A suspension containing 106 UFC mL-1 was incorporated into Müeller-Hinton agar (Himedia®). Agar wells (6 x 3 mm) were filled with 8 μL of concentrated solution of each extract (100 mg/mL). As a positive control, 10 mg/mL of cyclopirox olamine (Uci-Farma®) were used. After the overnight incubation period, the inhibition zone was measured with the aid of a millimeter ruler and diameters equal to or greater than 7 mm were considered valid (Nascimento, Locatelli, & Freitas, 2000).
Statistical analysis
The results of the inhibition coefficients (GI50) were analyzed using the GraphPad Prism® 5.0 program. The statistical differences between the experimental groups were detected by ANOVA and the values were compared with the Tukey test, with p < 0.05 for statistically significant differences. The comparison of treatments with the control group was made using the Dunnett test, with α < 0.05 for statistically significant differences. The effect of plant extracts and phytochemicals on tumor cells was evaluated using simple linear regression, analyzing the behavior of the curve in relation to the chemotherapeutic 5-Fluorouracil. Among the treatments, Pearson's correlation analysis was carried out to evaluate the chemotherapy in the accumulated 72 hour period.
RESULTS
Preliminary phytochemical prospecting of hydroalcoholic extracts
The yield of the dry extract of R. dolabripetala was 2.31%. The phytochemical prospection of hydroalcoholic extracts was evaluated and revealed the presence of phytocompounds flavonols, tannins and xanthones. These findings are consistent with some studies involving other annonaceae plants in which these phytochemicals are also present in different degrees as for Annona reticulate (Verma, 2020) and other annonaceae plants (Chowdhury, Tareq, Tareq, Farhad, & Sayeed, 2021; Gowsalya & Sathya, 2021; Kumar et al., 2021; Medeiros et al., 2021; Shehata, Abu-Serie, El-Aziz, Mohammad, & El-Sohaimy, 2021; Yathzamiry et al., 2021).
The phytocompounds flavonoids anthocyanidin, anthocyanin, flavanones and flavanonols, anthraquinones and saponins were not detected. These findings are consistent with some studies involving other annonaceae plants in which these phytochemicals were also not found or were detected in minimal quantities as for anthraquinones and saponins (Gowsalya & Sathya, 2021; Perrone et al., 2022; Verma, 2020) and flavonoids and anthocyanins (Pathak, Pendhari, & Gunjal, 2020). There was a discrepancy in some phytochemicals detected in R. dolabripetala compared to other species of the genus, as observed for tannins absent in R. laurifolia (Cunha et al., 2009). In contrast, experimental samples from different annonaceae organs reveal high concentrations of flavonoids (Andrade et al., 2021; Costa et al., 2021; Oliveira, Sánchez, Monteiro, & Medeiros, 2022; Pandiyan & Ilango, 2022; Perrone et al., 2022; Shehata et al., 2021; Silva et al., 2021) and saponins (G’massampou et al., 2021; Oliveira et al., 2022; Pandiyan & Ilango, 2022; Pathak et al., 2020).
In addition to the interspecific factor, these discrepant results may occur, among other issues, due to technical, ecological or physiological factors, even within the species under study (Afonso, Pisano, Silva, Scaminio, & Olivieri, 2015; Fernandez et al., 2018; Nendissa, Mahendradatta, & Bastian, 2021; Shehata et al., 2021; Villalva et al., 2021). Phytochemical diversity is related to several plant ecophysiological functions and seasonal variation of secondary metabolites in response to phenology and climate must be considered (Yeshi, Crayn, Ritmejerytė, & Wangchuk, 2022), as observed for tannins in Xylopia emarginata (Medeiros et al., 2021). Considering the extraction solvent technique used and the plant organ phytochemically analyzed, different results for the phytocompounds were obtained in experimental tests with annonaceae such as R. mucosa (Araujo et al., 2021), A. muricata (Andrade et al., 2021; Villalva et al., 2021; Yathzamiry et al., 2021), A. cherimola (Villalva et al., 2021), A. crassiflora (Ribeiro et al., 2018), A. squamosa (Kumar et al., 2021) and Huberantha senjiana (Pandiyan & Ilango, 2022).
Determination of the antioxidant activity of extracts
In the evaluation of antioxidant activity, methanol was used as a dilution solvent (60 μM) for both the experimental sample and DPPH. The unfractionated extract (5.0 μg/mL) of the R. dolabripetala leaf extract exhibited 22.82% ± 0.11, statistically significant in relation to the antioxidant activity of 36.70% ± 1.03 of the phytotherapeutic control Ginkgo biloba (Pharmanostra®). The lipid peroxidation method indicated 24.78% for R. dolabripetala; the controls showed antioxidant activities of 80.90% for BHT and 28.66% for G. biloba.
The bioactivity that antioxidants exert in the prevention of different types of cancer and other diseases related to oxidative stress is already well studied (Dana, Sadoughi, Asemi, & Yousefi, 2022; Snezhkina et al., 2019). Although the antioxidation results presented by the R. dolabripetala extract are in a relatively comparable range to the Ginkgo biloba plant extract standard, and even with the antioxidant power of flavonols (Saha, Panieri, Suzen, & Saso, 2020), tannins (Andrade et al., 2021; Sieniawska & Baj, 2017; Smeriglio et al., 2017; Zhang, Wang, Jia, Cao, & Zhang, 2021) and xanthones (Li, 2018; Pinto et al., 2021; Zhou et al., 2018) only the presence of these phytocompounds was not able to potentiate this bioactivity displayed in R. dolabripetala. Xanthonic compounds present in A. murica and Garcinia mangostana exhibit strong antioxidant potential as observed in injured liver cells (Adelia, Widodo, & Noor, 2019). Although antioxidant phenolics were detected in R. mucosa (Araujo et al., 2021; Fernandez et al., 2018) and other annonaceae (Andrade et al., 2021; Perrone et al., 2022; Rocha, Dutra, Lorenzo, & Almeida, 2021; Shehata et al., 2021), the antioxidant potential exhibited by R. dolabripetala is much lower than that shown by other species of Annona genus, with values ranging from 31 to 97% (Chowdhury et al., 2021).
In our experimental tests, analyses of phytocompounds such as alkaloids, present in many annonaceae (Chen, Chia, & Huang, 2021; Costa et al., 2021; Justino et al., 2021; Kumar et al., 2021; Pandiyan & Ilango, 2022; Perrone et al., 2022; Rocha et al., 2021) that also exert antioxidant activity, were not included. In A. muricata, the strong antioxidant effect is highly correlated, mainly, to phytochemicals such as flavonoids and phenolic compounds, however, several other antioxidant compounds have been identified, such as tannins, saponins, glycoside, and acetogenins in this species (Esparza & González, 2020; Wahab, Jantan, Haque, & Arshad, 2018) and other annonaceous (Andrade et al., 2021; Gowsalya & Sathya, 2021; Kumar et al., 2021; Perrone et al., 2022; Villalva et al., 2021).
Cytotoxicity test
Tests with the DMSO solvent were performed in relation to the negative control and the concentration was determined up to 0.5% (Figure 2). The isolated effect of the chemotherapeutic 5- Fluorouracil on the proliferation of B16F10 melanoma cells was evaluated (Figure 3).
Figure 2
Comparative assay of cells cultured in a medium containing the dimethyl sulfoxide solvent (dmso; positive control) (0.50% ± 0.06) in relation to the negative control (0.589 ± 0.0618). P = 0.1697. Dunnett test (α = 5%).
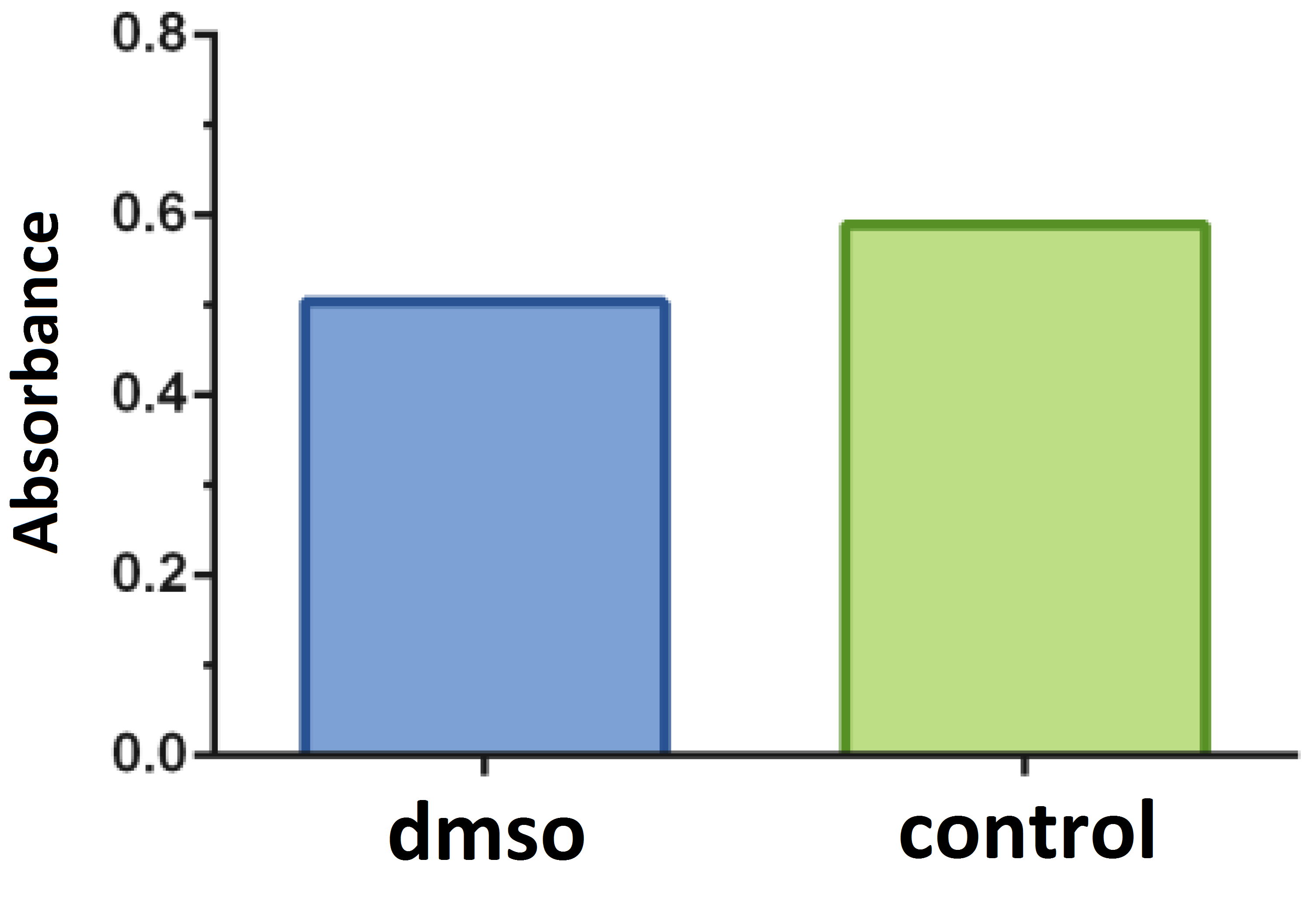
Figure 3
Linear regression plots of inhibition of the proliferation (%) of B16F10 melanoma cells exposed for up to 72 hours at different concentrations of the chemotherapeutic 5-Fluorouracil.
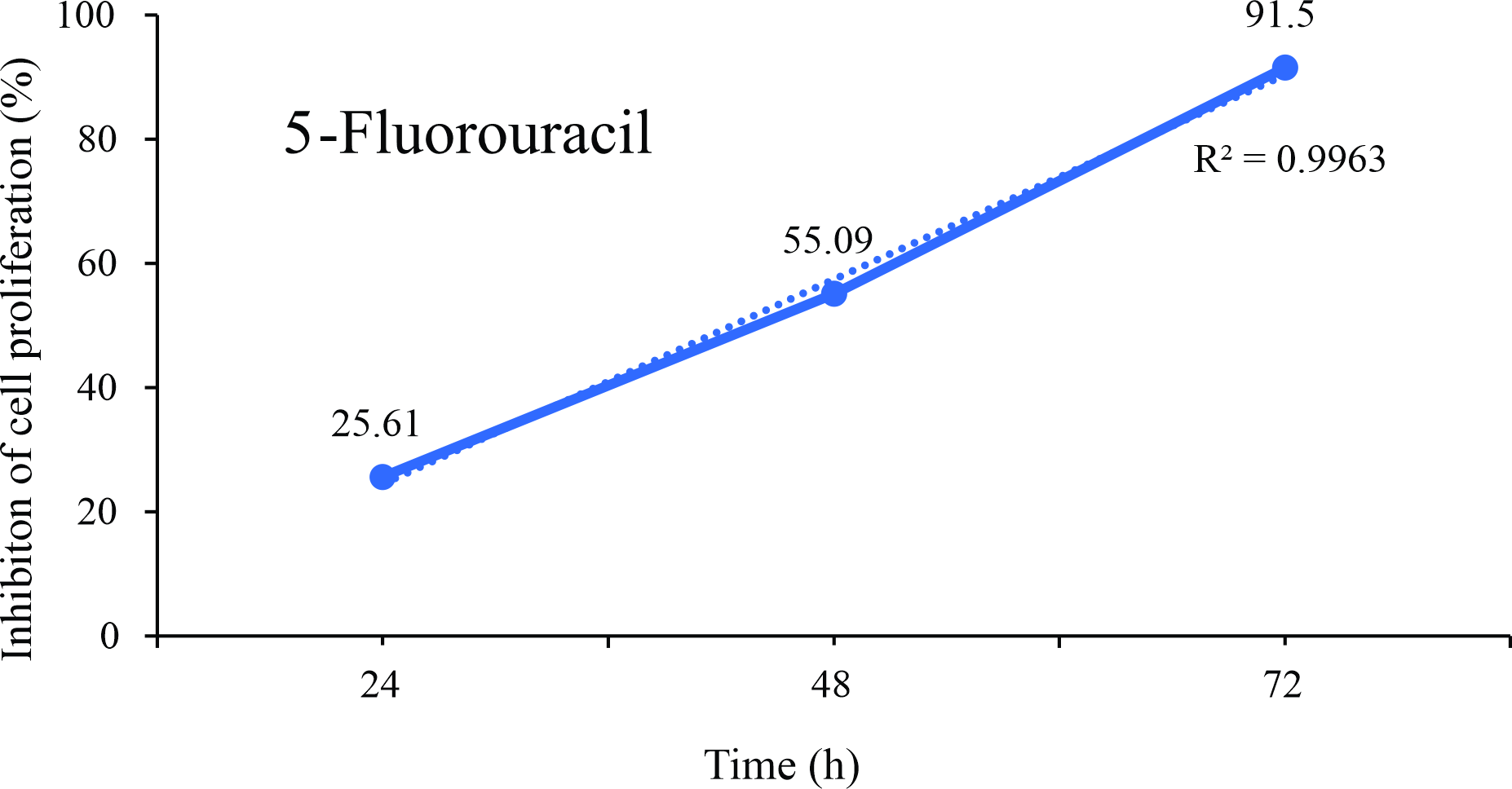
The antitumor activity of extracts and phytocompounds, expressed in the minimum lethal concentration (GI50), in the 72 hour period was evaluated (Figure 4) presenting the following results, in μg/mL: R. dolabripetala: 47.04 (Figure 4a), 1.7-dihydroxyxanthone: 0.98 (Figure 4b), xanthone: 3.38 (Figure 4c), 5-Fluorouracil: 0.24 (Figure 4d).
Figure 4
GI50 of the (a) R. dolabripetala leaf extract, (b) 1.7-dihydroxy-xanthone, (c) Xanthone and (d) chemotherapeutic 5-Fluorouracil, determined by non-linear regression analysis using the GraphPad Prism® 5.
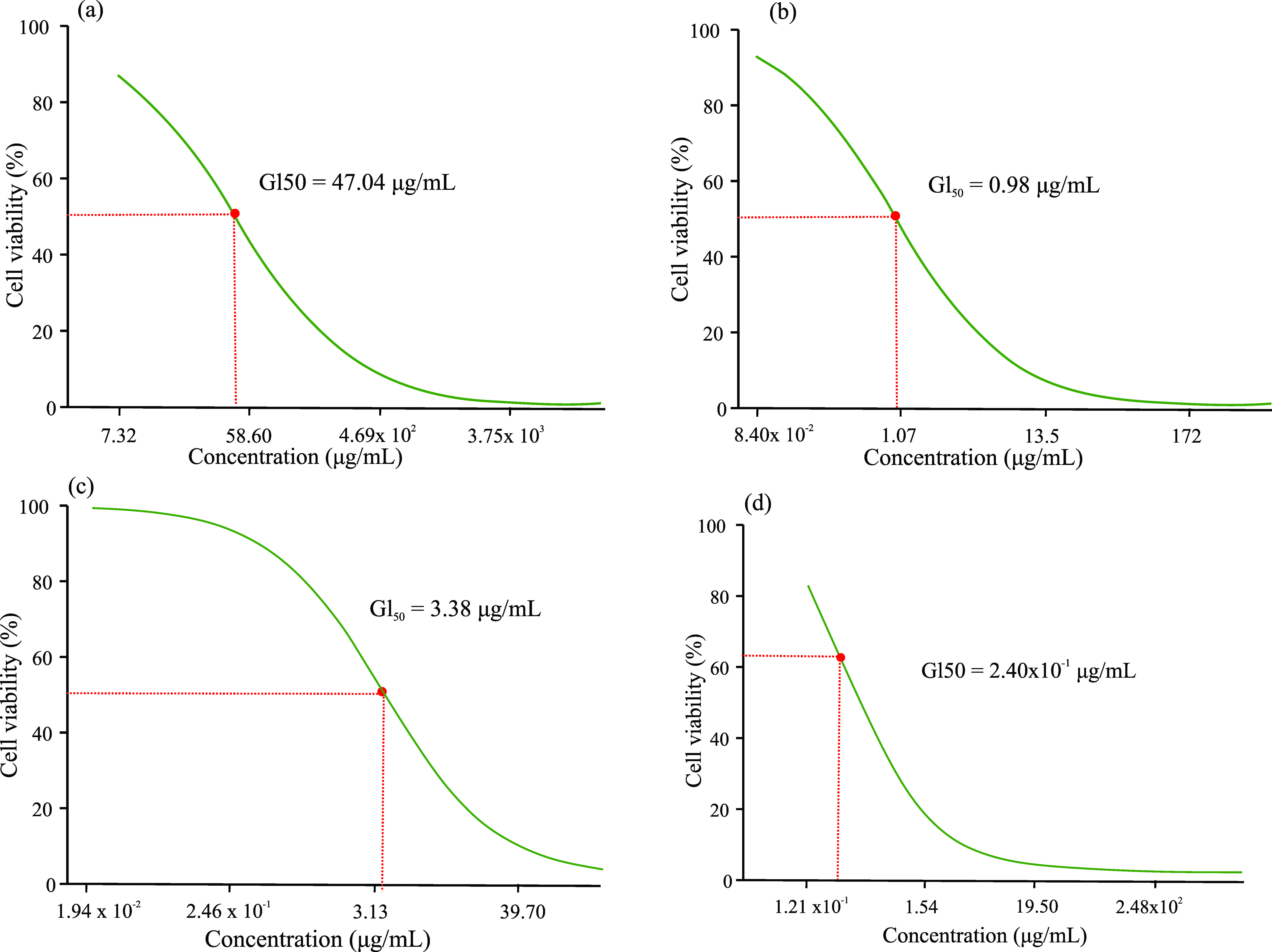
Xanthones polyphenols and their synthetic derivatives exhibit antitumor potential (Huang et al., 2021; Kurniawan et al., 2021; Pinto et al., 2021; Song et al., 2021; Song et al., 2022) and, interestingly, the treatment with RDLE that exhibits this phytochemical in its leaves, showed a very discrepant GI50. An investigation into a possible antagonistic effect among the extract's phytochemicals may clarify this result. Or even, the investigation of the cellular mechanism of the action of xanthones and derivatives in the tumor biochemical pathway may also clarify the specific action of this phytocompound at a point of carcinogenesis absent in melanoma.
By analyzing the GI50 of the treatments, new assays were carried out exposing B16F10 melanoma cells for up to 72 hours to four concentrations of the chemotherapeutic 5-Fluorouracil, RDLE and polyphenol 1.7-dihydroxyxanthone (Figure 5).
Figure 5
Linear regression plots of inhibition of the proliferation of B16F10 melanoma cells exposed for up to 72 hours at different concentrations of the chemotherapeutic 5-Fluorouracil (a), R. dolabripetala extract (b) and phytochemical 1.7-dihydroxy-xanthone (c).
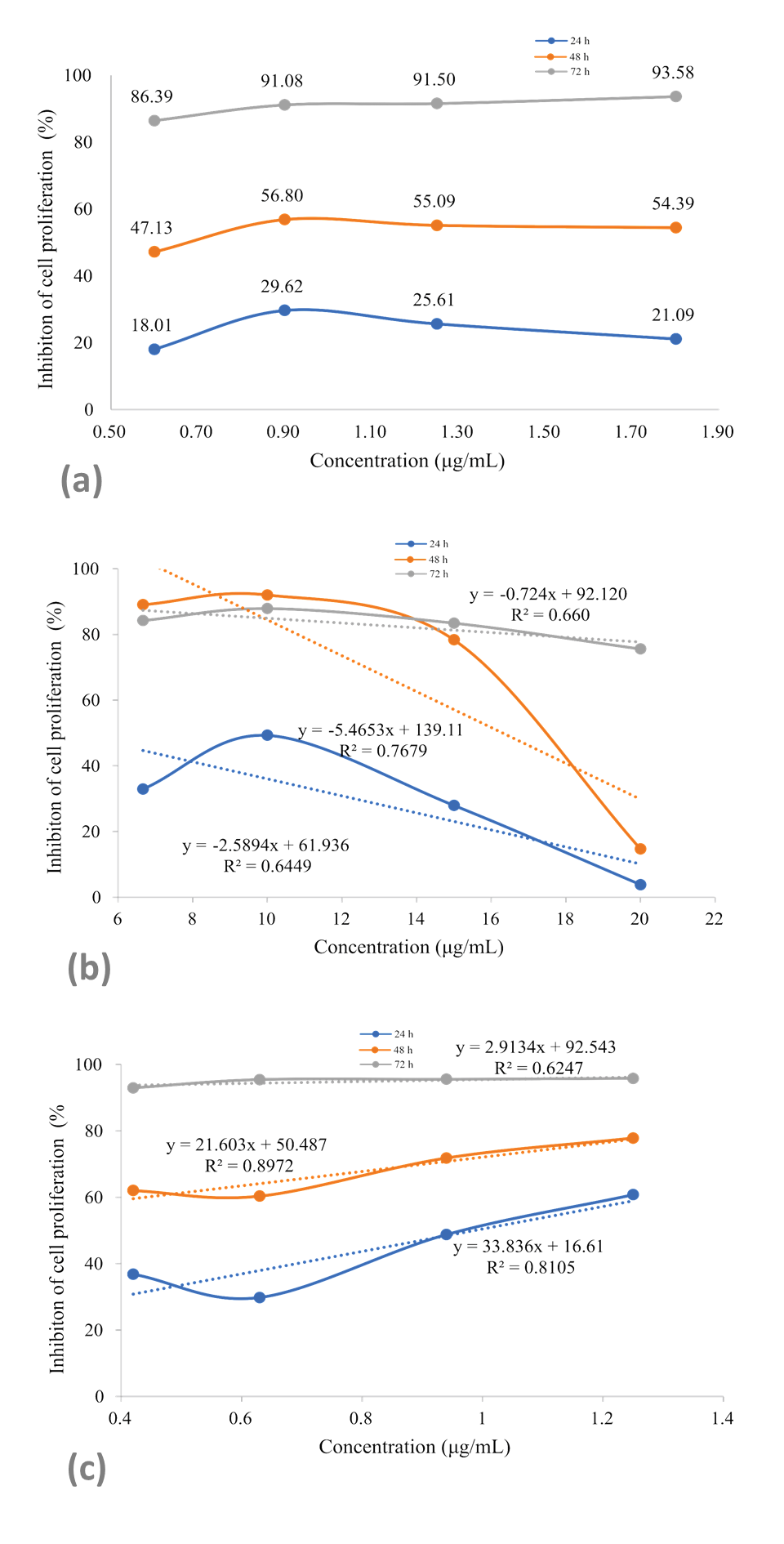
The R. dolabripetala treatment reduces the inhibition rate with increased dosage and, in low doses (6.67 to 10 x 103 μg/mL), the cellular inhibition rate is high, for up to 48 hours. Tumor cells exhibit proliferation inhibitory rates after exposure to 0.6 x 103 μg/mL of 1.7-dihydroxy-xanthone and after 72 hours, the dose increase has little influence. During this period, under the concentration of 0.4 x 103 μg/mL, the inhibition rate is 92% and is balanced at 95% with increased dosage. Xanthone derivatives (gambogic acid and α-mangostin) impair colorectal cancer proliferation, motility, adhesion to extracellular matrix and to endothelial cells, and also induce apoptosis and cell death (Rech et al., 2021).
When comparing treatments with 5-Fluorouracil, the best correlation between an increase in dosage and the inhibition rate occurs with 1.7-dihydroxy-xanthone. In the R. dolabripetala treatment, the correlation is high when the dose is increased (Table 1). Tests that can associate treatments with evaluation of the synergistic effect between them provide an interesting perspective, such as, for example, cell exposure to chemotherapy for 24 hours and immediately after a second exposure of cells to the flavonoid alone or associated with it. Xanthonic derivatives (gambogic acid and α-mangostin) exhibit comparative results to the chemotherapeutic agents cisplatin and 5-fluorouracil on colon tumor cells (Rech et al., 2021) and doxorubicin and vinblastine on leukemia, hepatocarcinoma and breast cancer cell lines (Kuete et al., 2014).
Table 1
Levels of correlation of each trial of the 5-Fluorouracil treatment (1.25 x 103 μg/mL) and for each treatment (R. dolabripetala, 1.7-dihydroxy-xanthone) and experimental doses (μg/mL)
R. dolabripetala | 1.7-dihydroxy-xanthone | ||
6.67 x 103 | 0.79 | 0.42 103 | 1.00 |
10 x 103 | 0.78 | 0.63 103 | 1.00 |
15 x 103 | 0.88 | 0.94 103 | 1.00 |
20 x 103 | 0.95 | 1.25 103 | 1.00 |
From the experimental results on cell proliferation, B16F10 melanoma cells were cultured for up to 72 hours at the concentration of each treatment that exhibited the greatest cellular inhibitory effect: RDLE: 10.0 x 103 μg/mL; 1,7-dihydroxyxanthone: 1.25 x 103 and 5-Fluorouracil: 1.25 x 103 (Figure 6).
Figure 6
Assay with B16F10 melanoma cells exposed for up to 72 hours to the most efficient inhibitory concentration of RDLE (10.0 x 103 μg/mL), of the phytochemical 1.7-dihydroxy-xanthone (1.25 x 103 μg/mL) and of the chemotherapeutic 5-Fluorouracil (1.25 x 103 μg/mL) (*) statistical difference in relation to the positive (5-Fluorouracil) control by the Dunnett test (α = 5%).
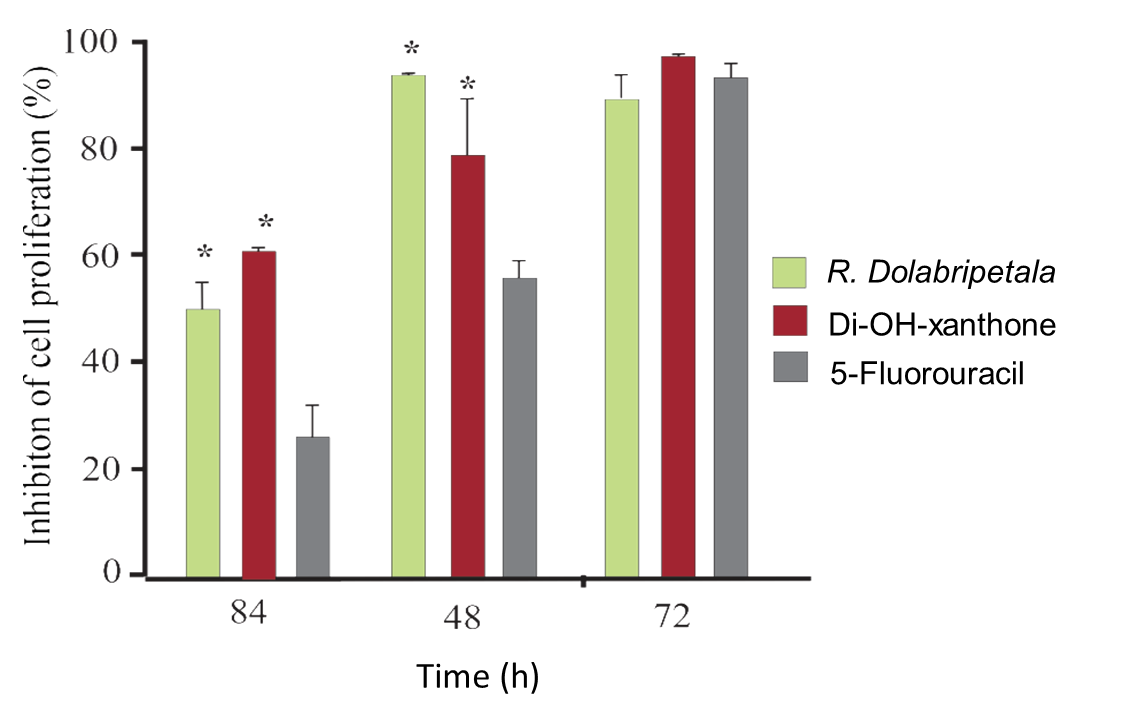
Xanthonic derivatives (such as α-mangostine, β-mangostine, γ-mangostine, gartanine, 8-deoxygartanine, garcinone C, garcinone D, 9-hydroxycalabaxanthone, mangaxanthone B, and 3-isomangostine) present in Garcinia (Clusiaceae) show effects on the viability of different tumor cell lines (Nguyen, Qu, Nguyen, Le, & Chen, 2022; Song et al., 2022; Zhao et al., 2022). The strongest inhibitors were α-mangostine and γ-mangostine, indicating that the position of the main functional groups, including hydroxyl and isoprenyl groups, are important in promoting anti-proliferative effects against prostate and breast cancer cells by inducing apoptosis through human estrogen receptors and fatty acid synthase signaling pathways (See et al., 2022), or through the inhibition of cyclin-dependent kinases (CDK2/CyclinE1), central regulators in tumorigenesis (Nauman, Tocmo, Vemu, Veenstra, & Johnson, 2021). Xanthonic phytocompounds also promote the interruption of the cell cycle at G2/M phase exhibiting strong antitumor cell proliferation and topoisomerase II inhibitory activity (Song et al., 2022) and between G0/G1 and S phases, strongly inducing apoptosis via caspases 3/7, 8 and 9 activation and disrupted the mitochondrial membrane potential in different cancer cells lines (Kuete et al., 2014). Temporally, cytotoxicity is more effective in treatments in relation to chemotherapeutic control, in which a larger population of melanoma tumor cells is eliminated when exposed to the most effective concentrations previously determined for phytocompounds and plant leaf extracts. With up to 72 hours of culture, there is no statistical difference between the assays with more than 90% inhibition of cell proliferation (Figure 6).
Plant-derived bioactive compounds, especially polyphenolic, have shown to reduce tumorigenesis, interfering in the initiation, promotion and progression of cancer (Dana et al., 2022; George et al., 2021; Hu et al., 2022) including melanoma (Enaru et al., 2021; Khan et al., 2020; Musial, Kroplewska, Kmiec, & Ponikowska, 2021; Patra et al., 2021; Pinto et al., 2021). Cytotoxic phytocompounds present in different species of the genera Rollinia (Araujo et al., 2021; Cunha et al., 2009) and other annonaceous (George et al., 2021; Handayani & Yuzammi, 2021; Khan et al., 2020; Perrone et al., 2022; Rocha et al., 2021; Shehata et al., 2021) support the experimental results obtained here, with selective cytotoxic effect on normal cells (Ribeiro et al., 2018; Shehata et al., 2021). The antitumor action of xanthones and derivatives (Huang et al., 2021; Kurniawan et al., 2021; Nguyen et al., 2022; Pinto et al., 2021; Song et al., 2021; Song et al., 2022; Zhao et al., 2022), and tannins (Musial et al., 2021; Shehata et al., 2021; Zeng, Jiang, Du, & Kokini, 2022; Zhang et al., 2021) also reinforces the data. Regarding the results exhibited by the phytochemical xanthone, new experimental tests need to be conducted to understand its cytotoxic action on cellular pathways as a function of the chemical groups (such as 1.7-dihydroxy-xanthone) present in this polyphenol.
In our experimental tests, as mentioned, analyses of alkaloids, phytochemicals present in many annonaceae, which also exert antitumor action as investigated in R. leptopetala (Costa et al., 2012), R. mucosa (Araujo et al., 2021), Diclinanona calycina (Costa et al., 2021), A. muricata (Wahab et al., 2018) and A. cherimola (Perrone et al., 2022), were not included. Another class of phytochemicals not included in our screening was acetogenins, cytotoxic phytocompounds, characteristic of annonaceae (Chen et al., 2021; Dev & Joseph, 2021; Lima, Faustino, Allahdadi, França, & Pinto, 2022; Rocha et al., 2021; Villalva et al., 2021) as found in R. mucosa (Araujo et al., 2021; Liaw, Chang, Wu, & Wu, 2003) and R. laurifolia (Cunha et al., 2009; Narwade & Aher, 2019; Nascimento, Boaventura, Assunção, & Pimenta, 2003). Annonaceous acetogenins constitute a series of polyketides found almost exclusively in plants of the Annonaceae family with bioactivity and mechanisms of action of scientific interest (Justino et al., 2021; Kazman, Harnett, Hanrahan, & R, 2022; Nascimento et al., 2022; Neske et al., 2020). The mechanism of cytotoxic action of acetogenins consists mainly in the inhibition of mitochondrial complex I electron transport resulting in inhibition of cellular respiration and selective cytotoxicity against cancer cells (Londershausen, Leicht, Lieb, Moeschler, & Weiss, 1991) and is explained by the metabolic specificity of tumor cells (Snezhkina et al., 2019; Warburg, 1956). Acetogenins isolated from A. pittieri and Cymbonopetallum costaricense showed cytotoxic activity against human leukemic cells with low activity against healthy blood cells (Parra, Ford, & Murillo, 2021). On the other hand, nanoparticulate extracts of A. muricata have been shown to be highly cytotoxic to macrophages, although they exhibited a strong antitumor effect on melanoma and other cancer cell lines (Pedroza et al., 2021). Furthermore, aqueous and ethanolic extracts of A. muricata did not show a cytotoxic effect on human cervical adenocarcinoma HeLa cells at any concentration tested, however, for the normal 3T3 fibroblast cell line, fractions of one of the aqueous extracts were cytotoxic for this cell lineage (Yathzamiry et al., 2021).
Antibacterial activity of extracts
The effect of plant extract compared to the ciclopirox antibiotic on Gram-positive strains SA13, SA75, SB01 was evaluated (Table 2 and Figure 3). Inhibition halos in agar diffusion cultivation with a diameter of 7 mm or more were considered valid (Nascimento et al., 2000).
Table 2
Inhibition halo (mm) in cultivation of three strains of Gram-positive bacteria S. aureus 29213, S. aureus 4075 and Streptococcus bovis by the action of R. dolabripetala leaf extracts. Values in mean ± standard deviation.
Strain | Ciclopirox amine(positive control) | R. dolabripetala |
S. bovis | 20.00 ± 0.10 | 0.00 |
S. aureus 4075 | 22.67 ± 0.58 | 0.00 |
S. aureus 29213 | 24.00 ± 1.00 | 7.00 ± 0.38 |
There was no antibacterial effect of the RDLE on SB01 and SA75 strains, although studies point to antistreptococcus (Hernández et al., 2021; Lall et al., 2017; Perez & Gomez, 2019) and antistaphylococcus (Chen et al., 2021; Gowsalya & Sathya, 2021; Hernández et al., 2021; Perrone et al., 2022; Villalva et al., 2021) activity of other annonaceae, including the same Staphylococcus strain ATCC® 29213 used in the experimental trials (G’massampou et al., 2021). There are discrepant experimental tests regarding the inhibitory potential against S. aureus of leaf extracts of A. muricata, with positive (Legi, Edy, & Abdullah, 2021; Tahir & Damayanti, 2021) and negative (Komalasari, Alkausar, & Retnaningsih, 2021) experimental results.
Detected in RDLE, tannins (G’massampou et al., 2021; Sieniawska & Baj, 2017; Smeriglio et al., 2017) and several xanthone derivatives (Huang et al., 2021; Pinto et al., 2021; Song et al., 2021; Yuanita et al., 2020) exhibit anti-Staphylococcal bioactivity. Leaf extracts of A. crassiflora that had these phytochemicals removed, and no inhibitory effects on bacterial growth were observed (Ribeiro et al., 2018). Different xanthonic derivatives (rheediaxanthone-B, isorheediaxanthone, forbexanthone, baphikixanthone) present in several plant species including annonaceae exhibit a strong potential to inhibit Glucosamine-6-phosphate synthase, an enzyme required for bacterial and fungal cell wall synthesis (Ogunwa, 2017). Xanthonic derivatives such as α-mangostin present in Garcinia mangostana and 1,3,5,6-tetrahydroxy-2-(3,3-dimethylallyl) present in Calophyllum brasiliense and Mammea americana (Clusiaceae) exhibit antibacterial activity, including Staphylococcus aureus and Staphylococcus epidermidis penicillin and methicillin-resistant (Pratiwi, 2021; Sundaram, Gopalakrishnan, Subramanian, Shankaranarayanan, & Kameswaran, 1983; Yasunaka et al., 2005). Alkaloids also exert antibacterial action including Staphylococcus (Chen et al., 2021; G’massampou et al., 2021; Marco et al., 2019; Perrone et al., 2022). The experimental evaluation of other R. dolabripetala botanical organs and even other species of the Rollinia genus may open new lines of scientific investigation, including new bacterial strains.
The timing of field work for the acquisition of experimental plant samples needs to be considered. R. dolabripetala leaves were collected during the spring. Investigative scientific studies involving edaphoclimatic and ecological aspects and their relationship with the phytochemistry of plant organs would provide interesting results. In addition to the interspecific factor, discrepant results may occur, among other issues, due to technical, ecological or physiological factors, even within the species itself (Afonso et al., 2015; Arshan, Magi, & Mishra, 2020). For example, depending on the phytochemical extraction technique, plant extracts can provide differentiated results in antimicrobial assays (Nendissa et al., 2021; Patel, Shrivastava, & Kumar, 2009; Sanusi, Yunusa, Hamza, Usman, & Makama, 2019).
Tests with crude extracts of plant organs provide good indications of the plant's bioactivity and the prospect of isolation of promising biocompounds for the development of new future antitumor drugs. However, there is a limitation of predicting whether the isolation of a certain R. dolabripetala biocompounds will present interesting experimental results if there is synergy between the components of the leaf extract. Another limitation is to safely predict which chemical class has most potentially interfered with the viability of the tumor cell. In addition, it would be promising to investigate the action points of phytochemicals isolated from R. dolabripetala in tumorigenesis, since there are different biochemical pathways for each type of cancer. This is the first investigative study on the antitumor bioactivity of RDLE, which somewhat limits the safe inference of the results obtained. On the other hand, it will contribute as a source of primary data for future scientific studies involving this annonaceae species. Further studies at our research center will be conducted in order to understand the chemical composition of fractionated bioactive substances present in the plant extract and their effects on carcinogenesis.
As discussed, phytochemicals can have powerful antitumor and antimicrobial effects. As phytochemicals can vary within the same plant species, scientific studies can be directed to investigate the interference of different environments in which R. dolabripetala is adapted; the histochemical variations and the relation to the phytochemical bioactivity on Staphylococcus bacteria; as well as other pathogenic microorganisms, melanoma and other human tumor cell lines. Tests with crude extracts of plant organs provide good indications of the plant's bioactivity and the prospect of isolation of promising biocompounds for the development of new future antitumor drugs. However, there is a limitation of predicting whether the isolation of certain plant biocompounds will present interesting experimental results if there is synergy between the components of the leaf and bark extracts. Further studies at our research center will be conducted in order to understand the chemical composition of fractionated bioactive substances present in the plant extract and their effects on other pathogenic microorganisms, on oncogenesis and also on normal human cells.
CONCLUSION
R. dolabripetala exhibits antioxidant, antistaphylococcal and antimelanoma bioactivities and 1.7-dihydroxy-xanthone exhibited the best correlation between an increase in dosage and the inhibition rate. Phytochemicals present in RDLE such as flavonols, tannins and xanthone reinforce the results obtained. Experimental trials need to be conducted to understand the antioxidant, cytotoxic and antimicrobial potential of xanthones in cellular pathways as a function of the chemical groups (such as 1.7-dihydroxy-xanthone) present in this polyphenol. The seasonal factor in the collection of plant samples may explain the discrepancies in the phytochemical data of R. dolabripetala compared to other species of the genus.
Funding
All authors gratefully acknowledge financial support received from CNPq, and CAPES (Ministry of Education, Brazil).
Author contributions
MGC, TTO, ASJ, MRC - Research concept and design, MGC - Collection and/or assembly of data, MGC, TTO, ASJ, MAF, CMPS, MRC - Data analysis and interpretation, MAF - Writing the article, TTO, ASJ, MAF, MRC, LMC - Critical revision of the article, All authors has approved the final version of the article.