Introduction
Glucose oxidase (β-D-glucose: oxygen-1-oxidoreductase or GOX) is an enzyme that catalyzes the oxidation of β-D-glucopyranose to gluconic acid using molecular oxygen as an electron acceptor with simultaneous production of hydrogen peroxide (or hydrogen peroxide) as shown in the metabolic pathway below (Figure 1):
Glucose oxidase (GOX) has a molecular weight between 130 and 175 kDa and specifically catalyzes the reaction involving the anomeric beta form of glucose. Its optimum pH is 5.5 and its isoelectric point (pI) is 4.2. The metabolic pathway involving this enzyme can be replicated in vitro and exploited as a biochemical technique for screening plants with anti-hyperglycemic properties (anti-diabetic plants) (Khang et al., 2011). In this case, it is sufficient to add to the reaction medium a peroxidase that catalyzes the reduction of hydrogen peroxide (H2O2) to water. The reaction is highlighted by the addition of a colorless chromogenic (secondary substrate) which is oxidized to a colored product whose intensity is proportional to the concentration of glucose in solution. Two chromogenic substrates are generally used. These are ABTS (2, 2'-Azino-di-(3-ethylbenzthiazolin-sulfonate) which can be oxidized by H2O2 to a bluish-green product absorbing at 420 nm; and O-dianisidine which can be oxidized to quinone-imine, a dye which absorbs at 500 nm (Mgbeje, Terungwa, Ugoanyanwu, & Ebong, 2016). For this purpose, hyper-glycemia in vitro (glucose ≥ 100 mg/dL) can be detected using UV-visible spectrophotometry. Thus, any plant extract which can reduce the intensity of coloration of the reaction medium and thus the concentration of free glucose in solution would be displayed anti-hyperglycemic or hypoglycemic properties (Khang et al., 2011). UV-visible spectrometry can thus be used as the best means of studying the interaction of natural substances with GOX and glucose.
In the case of glucose oxidase inhibition (decrease of the affinity of the enzyme for the glucose substrate and/or the speed of the reaction involving the enzyme), such natural substances that interact with GOX and glucose are called Glucophages, as they allow experimental reduction of the concentration of free glucose (signal intensity) in vitro (hypoglycemic effect). Accordingly, in the absence of an inhibitor or when the extract is not active, the intensity of the signal (coloration) is strong. By the other hand, in the presence of an active extract (inhibitor), the signal intensity is low, probably due to the sequestration of free glucose in the form of a ternary glucose oxidase-glucose-inhibitor complex. The hypoglycemic effect in vitro is thus expressed as the result of inhibition of the oxidation of glucose to gluconic acid by glucose oxidase. In other words, any GOX inhibitor is a potential anti-hyperglycemic or hypoglycemic agent. It has been reported in the literature that natural substances are generally mixed inhibitors of key enzymes of carbohydrate metabolism and have the property of decreasing their catalytic power and reaction speed. Two biochemical mechanisms could explain this behavior. First, the free enzyme would attach to glucose (substrate) and the enzyme-substrate complex (ES) formed would then bind the inhibitor to give ESI. In a second step, the GOX enzyme would attach to the inhibitor and the binary ES complex would bind the glucose substrate to form the ternary ESI complex (Mgbeje et al., 2016). The present study aimed to use glucose oxidase as a marker enzyme for the in vitro detection of the anti-diabetic activity of two medicinal plants used in traditional medicine for the management of diabetes. UV-visible spectrophotometry, used in this study to monitor the activity of GOX, is based on electronic delocalization (transition) which leads to absorption bands characterized by wavelength and intensity. The absorption of light in the visible region of the spectrum by the chromogenic substrate can be modified in the presence of GOX inhibitor. Studying the absorption spectrum of the chromogenic substrate (ABTS or O-dianividin) is a very easy and reliable way to detect the action of GOX inhibitors and therefore to identify compounds/extracts with anti-hyperglycemic or hypoglycemic properties (Sushil & Talambedu, 2012).
Material and Methods
In vitro study
Plant material and reagents
The reactants consist of a glucose solution 17 mmol/L (3 g/L), extracts of Lippia multiflora and Vernonia amygdalina, and the enzymatic reagent consisting of the mixture glucose oxidase 15 KUI/L, peroxidase 1.5 KUI/L, and phenol 77 mmol/L in 0.1 M Potassium phosphate buffer: 1.36 g KH2PO4; 2.28 g K2HPO4*3H2O; 100 mL distilled water (Merck pa) at pH 7.0.
Free glucose level evaluation
Glucose is oxidized by oxygen to gluconic acid, producing hydrogen peroxide H2O2. The latter is dosed using colorimetric method. In the presence of peroxidase, the peroxide oxidizes phenol to quinone-imine whose absorbance is measured at 505 nm. Briefly, ten μL of a glucose solution (3 g/L) are mixed with other 10 μL of plant extract at different concentrations. The blank consists of distilled water, while Gliben is used as positive control. The resulting mixture is incubated at 25 °C within 15 min. The determination of free glucose is measured after the addition of 1 mL of the enzyme reagent (Glucose oxidase 15 kIU/L, peroxidase 1.5 kIU/L, phenol 77 mmol/L, 0.1 M Potassium buffer pH 7.0.) at 505 nm.
In silico (Molecular Docking) studies
Protein preparation
The crystal structure of the protein used in this study was retrieved from the Protein Data Bank with PDB ID 1GAL, and imported into chimera for visualizing the binding domain and identifying the amino acids in the binding pocket. The survey of the binding-site was carried out with the reference of amino acid residues in the binding domain, as previously reported (Anshika, Meghna, & Neeti, 2017; Vidya et al., 2019). The hydrogen atoms were added to the protein in order to correct the ionization and tautomeric states of the amino acid residues. The water molecules were removed before the docking. Incomplete side chains were replaced using the Drunbrack rotamer library (Shapovalov & Dunbrack, 2011). In addition, the protein was subjected to energy minimization by applying the AMBER 14SB force field method, and AM1-BCC was used for other residues with a maximum number of 200 steps and gradient descent method at a RMS gradient of 0.02.
The optimized protein was saved in pdbqt format and imported to PyRx for molecular docking which was carried out by using Autodock Vina virtual screening tool (Trott & Olson, 2010).
Generation of ligand dataset
The selected phytocompounds from Lippia multiflora derivatives from various literature resources (Arthur et al., 2011; Avlessi, Alitonou, Sohounhloue, Menut, & Bessiere, 2011; Bagora et al., 2014; Bassole et al., 2003; Soro et al., 2016) were drawn using ACD/MarvinSketch (20.9). The 2D structures of the sketched ligands (compounds 1-9) are shown in Figure 2. Further, ligands were imported into ChemDraw to obtain 3D from 2D. The 3D ligands were optimized using Mercury software by conformer generation.
In vivo anti-hyperglycemic activity
The study was performed on an animal model consisting of 15 Swiss mice subjected to temporary hyperglycemia by gavage of a glucose solution (200 mg/mL). These 15 mice were divided into three groups or batches as follows: the first group of 5 mice for the negative control (physiological water), the second group of 5 mice for the positive control (glibenclamide 10 mg/kg), and the third group of 5 mice for the test with the L. multiflora infusion at a dose of 250 mg/kg body weight. Blood glucose determination was performed using an SD check glucometer on whole blood collected from the tail.
Results and Discussion
In vitro bioassay
Figure 6; Figure 5; Figure 4; Figure 3 show the evolution of the glucose level as a function of the drug dose (Gliben or plant extract).
These curves show that the drugs used exponentially reduce the concentration of free glucose in vitro (in vitro hypoglycemic effect); so these drugs have the effect of sequestering/complexing glucose in vitro.
The drug concentrations that reduce 50% of free glucose levels in vitro are shown in Table 1.
Table 1
Mean IC50 values for anti-diabetic drugs
Drugs | IC50 (mg/mL) |
Gliben (positive control) | 0.36 ± 0.04 |
V. amygdalina (aqueous extract) | 3.00 ± 0.54 |
V. amygdalina (ethanolic extract) | 2.00 ± 0.31 |
L. multiflora (aqueous extract) | 1.36 ± 0.09 |
From this table, it can be seen that Gliben, a standard anti-diabetic agent, has shown a hypoglycemic effect in vitro. Thus, the perfect agreement between the calculated curves and the experimental points allows validating the used method. Moreover, the in vitro anti-hyperglycemic activity of L. multiflora and V. amygdalina confirms the in vivo antidiabetic activity of these medicinal plants as previously reported (Mgbeje et al., 2016; Ugoanyanwu, Mgbeje, Igile, & Ebong, 2015). This allows the use of UV-visible spectrophotometry as a technique for screening anti-diabetic plants using GOX activity. The present study showed that L. multiflora is more active than V. amygdalina (IC50: 1.36 ± 0.09 mg/mL Vs IC50: 3.00 ± 0.54 mg/mL).
The Figure 7 shows the evolution of optical density as a function of glucose concentration.
Figure 7 shows that Gliben and L. multifora reduce optical density, reflecting the inhibitory effect of these drugs on glucose oxidase in vitro. Thus, glucose oxidase inhibitory drugs have anti-glycemic properties (Njeri, Eliud, & Orinda, 2017) .
The effect of L. multiflora on free glucose concentration as a function of time is given in Figure 8.
The Figure 8 shows that L. multifora has the effect of reducing the concentration of free glucose as a function of time. This hypoglycemic effect in vitro is dose-dependent.
The molar extinction coefficient of quinone imine, which is the oxidation product of the second substrate (chromogen), was determined by linear regression (Figure 10) and represents the slope of the line.
Figure 9
Glucose absorption spectrum (after enzymatic derivation) in the presence of Gliben (0.5 mg/mL) and L. multiflora (2 mg/mL)
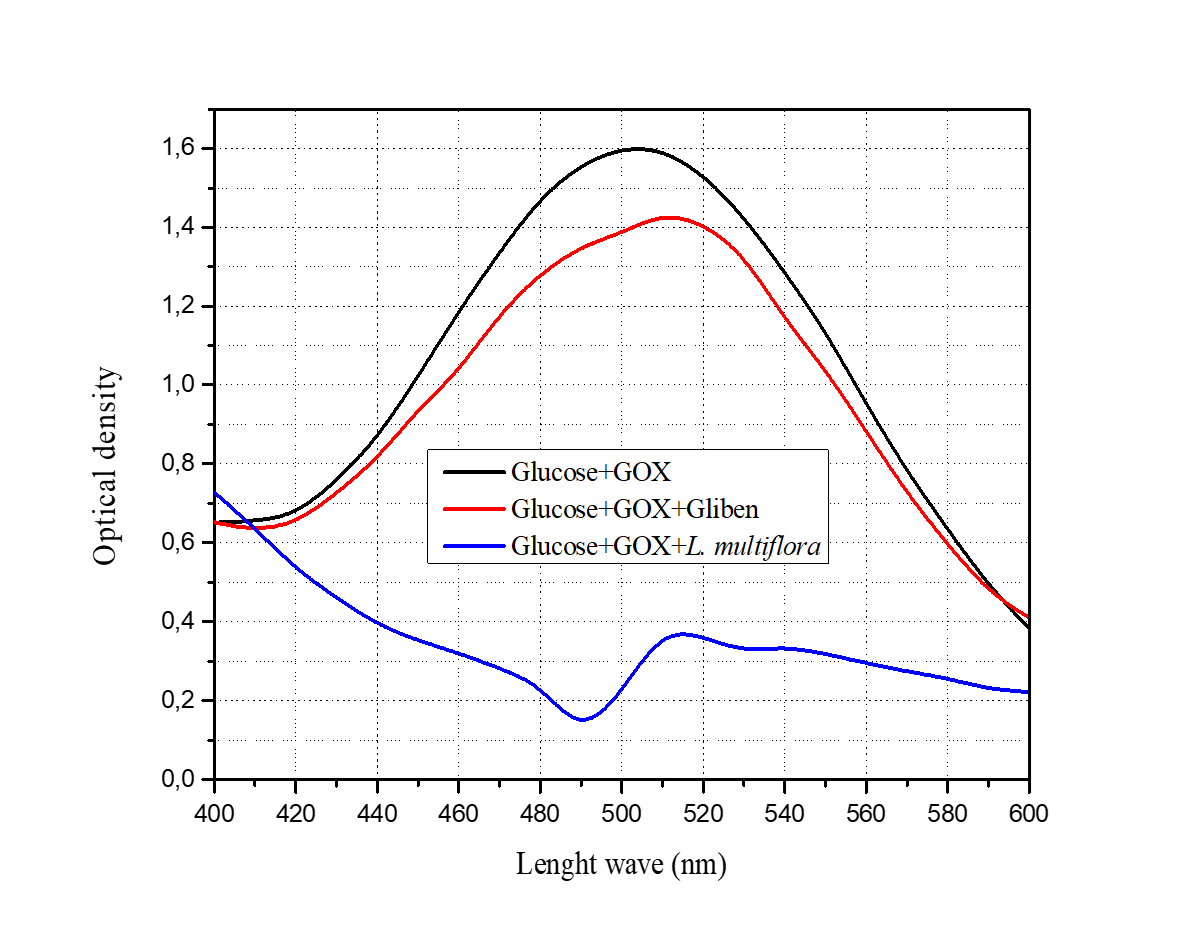
Figure 10
Calibration line for glucose in the presence of the enzyme reagent
(Glucose oxidase 15 kUI/L, peroxidase 1,5 kUI/L, phenol 77 mmol/L, pH 7.0., Potassium phosphate buffer, temperature 25 °C, Wavelength: 505 nm)
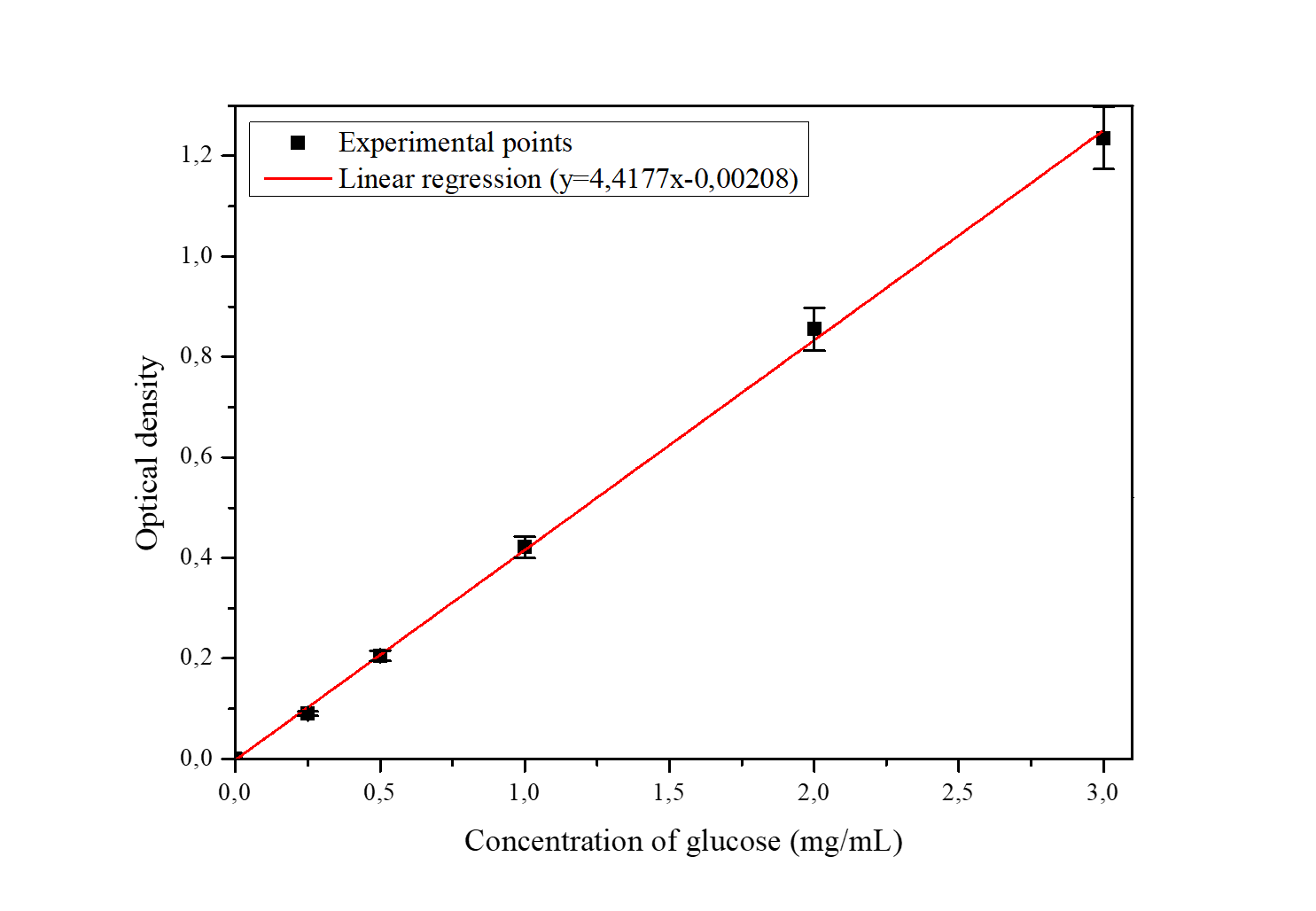
Knowledge of the molar extinction coefficient allowed the Michaelis-Menten equations to be determined in the presence and absence of glucose oxidase inhibitors. After linearization according to Lineweaver and Burk, the kinetic parameters were calculated (Table 2).
Table 2
Mean values of enzyme kinetic parameters in the presence and absence of the inhibitors
Table 2 shows that Gliben (0.5 mg/mL) and L. multiflora (2 mg/mL) reduce both the rate of oxidation of glucose by glucose oxidase (catalytic power) and the affinity of this enzyme for its substrate (glucose) via biochemical mechanism so called mixed inhibition. The present study revealed that Gliben, V. amygdalina, and L. multiflora decrease the concentration of free glucose in vitro. This hypoglycemic effect in vitro is manifested by a decrease in light intensity (absorbance) at 505 nm. These data confirm the results of previous work on the anti-diabetic properties of Gliben and the tested plants, thus validating the GOX/HRP enzyme system as a biochemical model for the in vitro anti-diabetic screening of medicinal plants (Ibegbu, Nnaemeka, Ikele, & Nwachukwu, 2018). The inhibition of enzymatic oxidation of glucose in vitro (expressed as glucose sequestration by the enzyme-drug complex or as enzyme-glucose-drug complex) is dose-dependent (Sushil et al., 2012). Thus, if glucose was not sequestered as an ESI complex, a decrease in light intensity at 505 nm, the absorption wavelength of the chromogenic substrate in its oxidized form, would not be expected. This study shows that de Gliben, V. amygdalina, and L. multiflora inhibit GOX from converting glucose to gluconic acid by reducing the concentration of free glucose (hypoglycemic effect) in vitro in the form of a GOX-glucose-drug complex (Figure 10). As diabetes is a major public health problem, the population is increasingly turning to herbal medicines for its management (Tunga, Kilembe, & Matondo, 2020).
The inhibitors of GOX activity are secondary metabolites present in active plants including Lippia multiflora.
Molecular docking studies
To determine the binding affinities and key interactions between 1GAL and L. multiflora compounds, docking studies were performed using Autodock 4.2. The binding pocket of the target protein was decided using a grid size of 50 x 50 x 50 Å3 with the help of an auto grid centered at x = 43.730, y = 12.702 and z = 59.371. Two important parameters were taken into account to select potential compounds from the given inputs: (i) the prediction of the binding energy of the best docking pose using the scores calculated by the Autodock scoring function and (ii) the details of the hydrogen bonds of the top ranked pose. The docking was run 10 times and average values for each compound were calculated. The summary of the docking information of the top ranked poses is presented in Table 3. To analyze the results, Glibenclamide was used reference molecule (positive control).
Table 3
Binding affinity energies of the tested phyto-compounds derived from L. multiflora
The Table 3 revealed that all the tested compounds form a thermodynamically stable complex with the GOX enzyme (1GAL). However, the best docked ligands are obtained with compounds 8 (-10.1±0.0 Kcal/mol), 6 (-9.5±0.1 Kcal/mol), 3 (-8.3±0.0 Kcal/mol), and 9 (-8.2±0.1 Kcal/mol). These results confirm the enzymatic inhibition mechanism proposed to explain the anti-diabetic activity of Lippia multiflora. However, it should be noted that only compounds 8 and 6 involve hydrogen bonds in the formation of the complex with the enzyme 1GAL: compound 8 forms five hydrogen bonds (ASN514, ASP424, ARG95, TYP68, LEU65) while compound 6 forms two hydrogen bonds (ASN514 and SER422).
However, no H-bonding interaction occurs in the complex that involves ligands 9 and 3 despite their high binding energy (-8.2±0.1 Kcal/mol and -8.3±0.0 Kcal/mol respectively). Similar results were also previously reported (Kasende, Matondo, Muya, & Scheiner, 2017; Mpiana et al., 2020). Indeed, several forces contribute to the stability of a complex between a receptor and a ligand, among them dispersion forces, mainly in the stacking interactions between aromatic systems, and van der Walls interactions (Scheiner, 2020) . The stabilization of complexes is strengthened by other interactions (π-cation, π-alkyl, π-sigma) as well (Matondo et al., 2021).
The results obtained in this study show that the GOX enzyme is inhibited by medicinal plant extracts according to the same mechanism of action as the enzymatic models traditionally used to evaluate the anti-diabetic activity of plants (Yusaka & Kuniyo, 2015). Thus, this enzyme can validly constitute a very good model for in vitro studies. The UV-visible spectroscopy used in this study is a physical method that allows high accuracy, and the standard spectrophotometer is an easy-to-handle device. Its relatively high purchase cost is compensated by the use of less expensive consumables. The use of this enzymatic test instead of animal models (mice, rats, guinea pigs) makes the method less expensive and quick for large-scale screening.
Indeed, diabetes is a real public health problem. According to the World Health Organization, about 80% of rural populations living in developing countries rely on Traditional Medicine to meet their health care needs. Hence, developing a simple but robust method for the scientific validation of herbs used for the management of diabetes may help to tackle the disease (Ngbolua et al., 2011; Ngbolua et al., 2011).
The results of the in vitro and in silico anti-diabetic activity using GOX enzyme as a model were validated by the in vivo study. Indeed, the in vivo study showed that L. multiflora reduced blood glucose levels very significantly in treated mice as shown in Figure 13.
The enzyme glucose oxidase plays an important role in basic research as a biosensor (Hassan, Vyas, Grieve, & Bartolo, 2021; Hyo, Ki, & Hyun, 2020; Stefano, Katsuhiro, & Koji, 2011). In this study, this enzyme was used to develop a UV-visible spectrophotometric method for screening plants with anti-diabetic properties.
Conclusion and Suggestions
The present study aimed to create hyperglycemia in vitro in order to exploit biochemical pathways involving glucose oxidase as an alternative to screen by UV-visible spectrophotometry medicinal plants for their anti-diabetic activity. The in vitro and in silico results revealed that glucose oxidase can serve as a marker enzyme for in vitro anti-diabetic activity evaluation of medicinal plants. GOX displays similar pharmacological sensitivity such as standardized glucosidic metabolism enzyme alpha amylase towards anti-diabetic drugs/plants (Glibenclamide, Lippia multiflora, and Vernonia amygdalina) through mixed inhibition. We suggest the use of this in vitro technique for the scientific validation of the bioactivity of plants species traditionally used for the management of diabetes.
Author contributions
Conceptualization: K.N.N., V.M. and P.T.M.; Methodology: C.M.A. and A.T.M.; validation, D.S.T.T., J.K.T., A.M. and K.N.N.; Formal analysis: C.I.L., B.G.Z. and L.M.E.; Investigation: C.M.A. and A.T.M.; Resources: D.T.D.; Data curation: J.K.T., A.M. and D.S.T.T; Writing-original draft preparation: C.M.A., A.T.M.; Writing-review and editing, J.T.K. and K.N.N; Visualization: C.M.A. and A.T.M.; Supervision: K.N.N.; Project administration: P.T.P. and V.M. All authors have read and agreed to the published version of the manuscript.