Introduction
Ionizing radiation (IR) is naturally or artificially the energy sort that is made up of electromagnetic radiation waves (X-rays either γ - rays) as well as molecules (beta, neutrons, and alpha). It can be created naturalistic or synthetically (Otto, 2010). Ionizing radiation has been increasingly utilized in medical imaging in x-rays, CT scans, nuclear medicine examinations, and interventional procedures. Higher or lower amounts from IR may affect human cells (Taqi, Faraj, Zaynal, Hameed, & Mahmood, 2018); the radiation interacts with human cells, whether directly by DNA or not directly by the highly productive and chemically unstable Reactive oxygen species (ROS). The indirect action occurs when the molecules of water in the cell are ionized by radiation, causing radiolysis of water resulting in free radical formation (Hertel, 2009). Free radicals are essential in our body's normal metabolic processes, including wound healing, pathogen removal, and tissue repair (Dong et al., 2020). However, regular exposure to low doses of IR in the hospital workers (Gao, Dong, Liu, Zhang, & Ao, 2020) may result in oxidative stress due to the dominance of excessive ROS formation over the antioxidant defenses (Nilsson & Liu, 2020), Which results in developing cancer and cardiovascular diseases (Nagane, Yasui, Kuppusamy, Yamashita, & Inanami, 2021). Lipid peroxidation, extraction for thiol groups from the membrane and cellular proteins, breaks in strands are considered the most significant effect of IR (Löbrich et al., 2005), and base alterations are the considerable consequences of ROS formation and eventually lead to DNA damage and cancer initiation (Fischer, Seo, & Efferth, 2018). A number of the foremost popular ROS (free radicals) are superoxide ion (O2), hydrogen peroxide (H2O2), and hydroxide radical (the most damaging OH). High concentrations of those components may promote carcinogenic activity (Seifried, Anderson, Fisher, & Milner, 2007).
Ionizing radiation is commonly used in diseases diagnosis and advanced therapeutic procedures in radiology and nuclear medicine. However, there is a high potential risk that faces workers utilizing these technologies, especially for those who do not use radiation protection tools (Ahmad et al., 2019; Stokke et al., 2017; Taqi et al., 2018). So, Periodically monitoring for DNA damage, oxidative stress, and immune response ranges for radiation-exposed workers is essential to guide health promotion and disease prevention (Ahmad et al., 2019). Many recent studies have raised concerns about the importance of utilizing natural antioxidants against the oxidative stress induced by IR (Dowlath et al., 2021; Musa & Shabeeb, 2019). Antioxidants may be endogenously produced internally by metabolism; moreover, they could be enzymatic and nonenzymatic, such as (melatonin, glutathione) or exogenous (dietary) antioxidants that cannot be made in the body and must be provided through foods or supplements (Niu & D, 2020). Dietary antioxidants like β carotenes, vitamins E and C, also mineral selenium exhibit long-term safeguarding, encompassing protection of post-irradiation towards deadliness and mutagenesis (Weiss & Landauer, 2003). That is because an action of antioxidants like "scavenging free radicals" through prohibiting and remedying damages indeed with ROS can thus strengthen the innate immunity and reduce the cancer risk and degenerative diseases (Lobo, Patil, Phatak, & Chandra, 2010). Regard as the 1st line defense toward damage of free radical (Hayes & McLELLAN, 1999) which consider as a new generation 'superheroes' to maintain the health (Aruoma, 1994). Antioxidants function as "free radical scavengers" by blocking and fixing harm inflicted from ROS and RNS. As a result, they can improve the immune system and reduce the risk of cancer and degenerative disorders (Lobo et al., 2010). It's also crucial in the prophylactic effect exerted by plant foods (Eskin & Shahidi, 2012; F, 1990; Gey, Puska, Jordan, & Moser, 1991; Liyana-Pathirana, Shahidi, & Alasalvar, 2006). Hence, this paper focuses on (discuses) the preventive role of dietary antioxidants against the harmful effect of IR among medical radiology workers.
Occupational ionizing radiation exposure
The risk that rises due to daily ionizing radiation exposure is still low, but health risks arise from cumulative exposure over a long time (stochastic effects) (Taqi et al., 2018). Several factors determine the biological effects of IR; The type of radiation used is one of them, the total dose, frequency of repetitive exposures, age, gender, and style of the target (Hertel, 2009) (organ) as there are variations in the radio-sensitivity between different cells and tissues (Shafiee et al., 2016). The radiation exposure time also has a significant role in increasing the effects, particularly at low doses (Taqi et al., 2018).
All requirements defined in the international protection guidelines against Ionizing radiation and the safety of radiation sources must be followed and applied by occupational workers exposed to radiation due to their work (Mora & Acuña, 2011). Estimating the doses received by occupationally exposed workers is also essential as it's a critical factor in government and organizations assessing radiation risks, establishing protective measures, and evaluating the parameters relating to the total radiation burden (Charles, 2001; Shoshtary, Islamian, Asadinezhad, & Sadremomtaz, 2016). As stated in its report number (103), the International Commission on Radiological Protection (ICRP) recommends that radiation doses for medically exposed workers be kept below the acceptable limits of 20 mSv/ year, with the additional provision that the effect does not surpass 50 mSv in any given year (ICRP, 2007). Although occupationally exposed to IR generally falls within the ICRP's Recommendations' Limits (less than 50 mSv) (ICRP, 2007), several investigations of workers exposed to IR have previously shown an increased risk of cancers such as leukemia and multiple myeloma (Rajaraman et al., 1994).
Free radicals
Free radicals are created when a chemical bond is broken so that each fragment retains one electron, when radicals are cleaved to produce new radicals, and when redox reactions occur (El-Masry & Mahmoud, 2021). Aerobe organisms can produce free radicals during the formation of ATP (adenosinetriphosphate) in mitochondria due to oxygen-reactive species, hydroxyl (OH) radicals and superoxide anion (O-2) are generated because of electron leakage through electron-transport steps of ATP production from mitochondria resulting in hydrogen peroxide (H2O2) development, more hydroxyl radicals are generated because of this reaction. They help us understand disease mechanisms because the free radical formation inside the cell causes a disproportion between antioxidant and pro-oxidant molecules. Lipids, DNA, and proteins oxidation will eventually lead to cell death. (Flora, 2007). Indeed, excessive amounts of free radicals and ROS produce oxidative stress and damage, which is associated with pathophysiology, development, and an indication of various diseases (Rabie, Tantawy, & Badr, 2016). Concurrently, it can cause lethal-chain reactions that deactivate essential proteins, enzymes, and other essential sub-cellular components required for cell survival following cell death. Therefore, enzymatic and non-enzymatic reactions that scavenge free radicals play a significant role in removing free radicals.
Reactive oxygen species (ROS)
ROS are oxygen-containing molecules with higher reactivity than molecular oxygen in the ground state. O2- and HO- are highly unstable. In others, H2O2 is widely available and has a long life span when a single electron is introduced to a ground state oxygen molecule, the superoxide radical.
Endogenous sources, enzymatic and non-enzymatic, generate ROS and external sources such as ionizing radiation. NADPH oxidase, found on the cellular membrane of polymorphonuclear cells, macrophages, and endothelial cells, is an example of an enzymatic source (Bm, Lambeth, & W., 2002; Vignais, 2002) and cytochrome P450-dependent oxygenase's (Coon, Ding, Pernecky, & Vaz, 1992). There are other sources of O2∙ − and H2O2 manufacture such as the proteolytic transformation of xanthine dehydrogenase to xanthine oxidase (enzymatic source), The direct transformation of single electrons to oxygen (O2) reduced coenzymes or prosthetic groups (e.g., flavins or iron Sulphur clusters) or the xenobiotics decline by certain enzymes are examples of non-enzymatic O2 processing (e.g., the anti-cancer agent Adriamycin or the herbicide-paraquat).
The medical radiology staff is susceptible to a high amount of exogenous ROS, disrupting the balance between antioxidant defenses and ROS production (Betteridge, 2000). Identified as oxidative stress plays a substantial role in many clinical conditions and malignant diseases, human immunodeficiency virus (HIV) infection, diabetes, atherosclerosis, and chronic inflammation, classified into two categories (Pantel et al., 1995). The commonly pro-oxidative change in the systemic redox state of thiol-disulfide and impaired glucose clearance of diabetes mellitus and cancer inferring that skeletal muscle mitochondria might be the primary site of higher ROS production. These circumstances are recognized as "mitochondrial-oxidative stress" (Kukielka et al., 1994). While the rest of the diseases are generally associated with increased NADPH oxidase activity caused by cytokines or other agents, which refer to "inflammatory oxidative conditions." Increased ROS or intra-cellular glutathione changes are related to pathological changes in signal cascades and dysregulation of gene expression, as demonstrated by cell adhesion molecule expression (Thiery et al., 1996).
Oxidative stress
Oxidative stress considers an essential subject of concern. It has shown that the development of various diseases is linked to oxidative stress, according to increasing evidence from studies on several diseases (Alghazeer, Alghazir, Awayn, Ahtiwesh, & Elgahmasi, 2018). The effects of hydrogen peroxide (H2O2), one of the most common free radicals, have been demonstrated to be similar to those of ionizing radiation in causing oxidative stress. The primary cause of radical hydroxyl damage is H2O2. The quantity of hydroxyl radicals in the body is partially controlled by the cellular antioxidant status (Jonas & Riley, 1992). Cells have their antioxidant types of machinery, which consist of several Phase II detoxification enzymes like glutathione peroxidases (GPXs) and the three superoxide dismutase isoforms (SOD1, SOD2, SOD3) (Masutani & Thioredoxin, 2002). This peroxidase's activity is dependent on the accessibility of reduced Glutathione. Regeneration of GSH from Glutathione disulfide (GSSG) by glutathione reductase includes NADPH as the electron-donor species (Riley, 1994). The preservation of intracellular redox homeostasis is dependent on the antioxidant system functions (Guo et al., 2019). Endogenous free radical reactions can lead to tumor formation, such as those induced by ionizing radiation (Lobo et al., 2010). Signal transduction, gene transcription, and the regulation of soluble guanylate cyclase activity in cells all depend on oxygen radicals (Lander, 1997; Zheng & Storz, 2000). Antioxidants, such as ascorbate acid (Mathew, Burritt, Mclachlan, & Pathirana, 2019), glutathione (Diengdoh, Kumaria, & Das, 2019), mitoquinone (Sui, Fan, Wang, Wang, & Chang, 2018), salidroside (Alotaibi, Slater, & Rahmoune, 2016), resveratrol, and so forth, can resist oxidative stress and reduce the damages from ROS.
Lipid peroxidation
In the lipid peroxidation process, the free radical absorbs electrons from cell membrane lipids, causing cell damage (Ateş-Alagöz, Coban, & Suzen, 2005; Niki, Yoshida, Saito, & Noguchi, 2005) which produces fatty acid radicals and also lipid hydroperoxides (LOOH) (Burton & Traber, 1990). Membrane structure and function are reversibly altered by lipid hydroperoxides (Girotti, 1998). Also are a source of high-reactive aldehydes that can modify DNA and proteins (Toyokuni et al., 1994), causing mutagenic and genotoxic effects (Hossain, Hosokawa, & Takahashi, 2008).
Lipid peroxidation causes cell membrane damage that is the molecular base of DNA for the turmoil of gene expression in signal transduction, and a set of cell functions related to apoptosis, acclimation, and the variability of genomic, all of which are firmly correlated with carcinogenesis (Loft & Poulsen, 1996; Trosko & Inoue, 1997) as well as a reduction in membrane fluidity and receptor alignment and the possibility of cellular lysis (Weydert, 1987). According to many studies, Malondialdehyde (MDA) levels are elevated in hospital workers' blood, particularly radiology staff, due to chronic low-dose ionizing radiation exposure (Rahzani et al., 2013). Malondialdehyde (MDA) is a lipid-peroxidation product produced when free radicals oxidize polyunsaturated fatty acids in membranes. It's also an oxidative damage biomarker (Ahmad et al., 2015).
Ionizing radiation and mitochondrial function
Mitochondria are essential for other cellular processes, such as synthesizing fatty acid, haem, and iron-Sulphur proteins and Ca2+ homeostasis (Tait & Green, 2012). Mitochondria are the only organelles other than the nucleus that have their DNA (Larsson & Da, 1995). Mitochondrial DNA comprises 13 genes that code for the components of the electron transport chain enzyme complexes (Complex I, II, III, and IV) and ATP synthase (Mcbride, Neuspiel, & Wasiak, 2006). The mitochondrial electron transport chain (ETC) has been extensively researched as a ROS source (Dalton, Shertzer, & Puga, 1999).
Stress-induced by exogenic factors such as ionizing radiation creates a nonspecific alteration of proteins, nucleic acids, and lipids within the mitochondria. These nonspecific alterations may cause mitochondrial damage and change mitochondrial function (Bulteau, Szweda, & Friguet, 2006). Any damage to mitochondrial function causes additional ROS production, exceeding the organelle's antioxidant potential. Un scavenged ROS can cause further destruction to mitochondrial components, including mitochondrial DNA (Okunieff et al., 2008). Several harmful changes in the circular mitochondrial DNA can be induced by Ionizing radiation, including strand-breaks, base-mismatches, and massive deletions, which might also be discovered in nuclear DNA (Wu et al., 1999). Hence, in addition to the cell nucleus, ionizing radiation may be directed primarily at mitochondria (Azimzadeh et al., 2011; Azzam, Jay-Gerin, & Pain, 2012) and a key target for ROS (Yakes & Houten, 1997).
(Richter, Park, & Ames, 1988) used isolated mitochondria from rat livers and directly irradiated them by the usage of γ-radiation (150 Gy). They discovered that mitochondrial DNA had six times more 8-hydroxydeoxy-guanosine (oxidized-base) per unit mass than nuclear DNA from the same liver (Richter et al., 1988) and this because: (1) the proximity of mtDNA to the site of ROS/RNS synthesis; (2) mtDNA histones and proteins are missing (which may shield it from oxidative damage) and (3) Because mitochondrial polymerases lack specificity for base excision repair and are altered by ROS, changes in polymerase function and increased mtDNA mutation rates may occur (Larsen, Rasmussen, & Rasmussen, 2005).
Effect of free radical on DNA and chromosomal aberrations
The cell division rate is inversely proportional to the number of lipid peroxides present in cells; the greater the degree of lipid peroxidation in cells, the slower cell division degree (Das, 1993). In hepatomas, the smaller the microsomal phospholipids level, the faster the tumor grows (Cheeseman, Burton, Ingold, & Slater, 1984; Das, 1993) have also reported that the lower level of lipid-peroxidation in hepatoma cells appears to be probably a mixture of factors, containing minimal quantities of polyunsaturated fatty acids, cytochrome P450, and alpha-tocopherol levels in lipid-soluble antioxidants.
Figure 1
The ionizing radiationresults in oxidative damage to DNA that causes changes in its structure and chromosomeaberrations such as translocation and deletion.
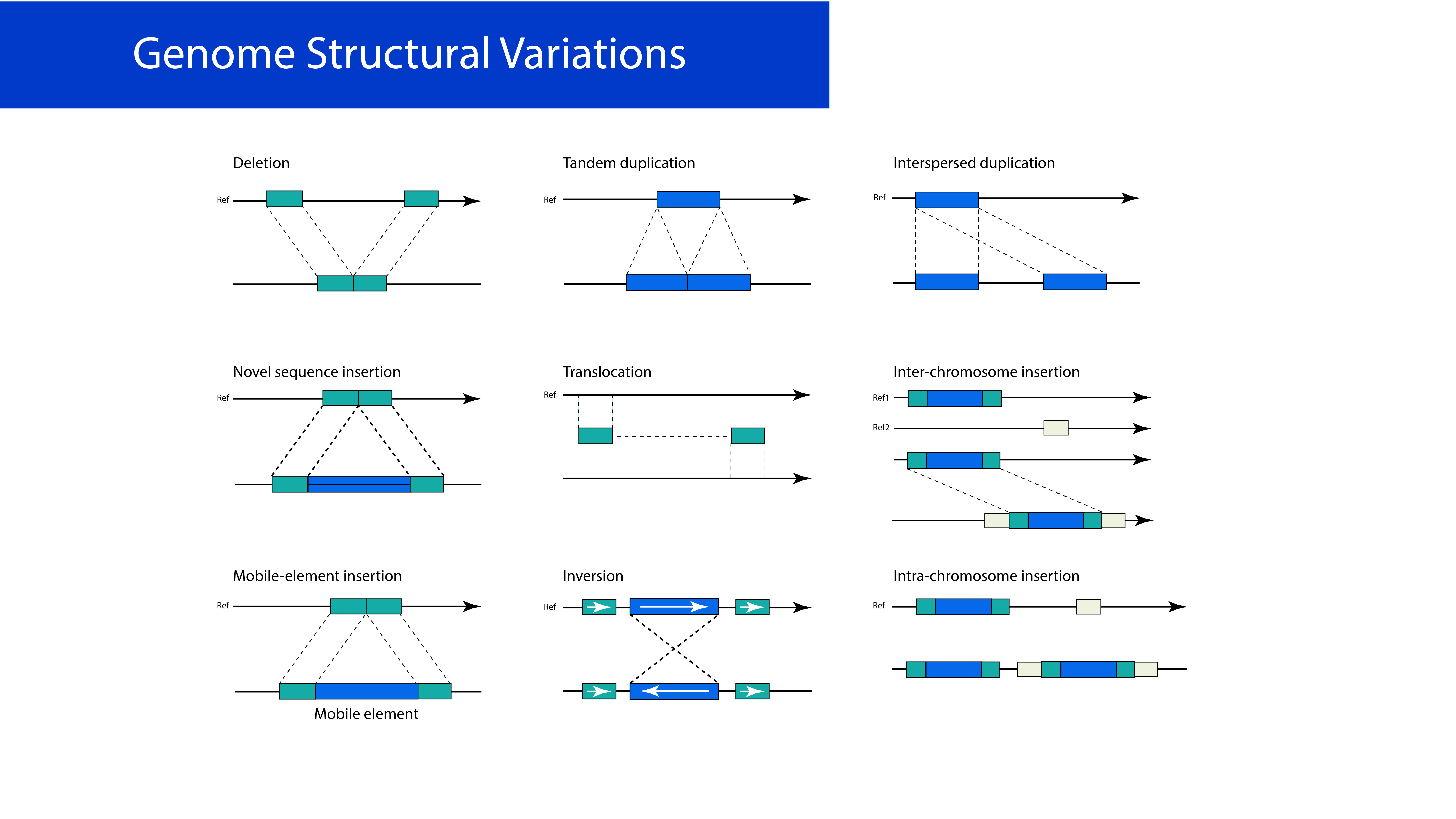
Eukaryotic cells' exposure to long-term IR results in polymorphisms in DNA repair genes (Aka, Mateuca, Buchet, Thierens, & Kirsch-Volders, 2004; Milić et al., 2015; Shkarupa, Henyk-Berezovska, Vo, Talko, & Klymenko, 2015) and oxidative damage to bio-molecules like DNA that causes changes and mutations in its structure (Figure 1). Also, the longest time the workers have been exposed to IR, the higher incidence of cancer (solid cancers and leukemia) (Sancar, Lindsey-Boltz, Ünsal-Kaçmaz, & Linn, 2004) and DNA breakage frequencies (Balakrishnan & Rao, 1999; Bonassi et al., 1997; Cardoso et al., 2001; Chung, Ryu, Kim, & Ha, 1996; Jacob et al., 2009; Maffei et al., 2004; Rozgaj, Kašuba, Šentija, & Prlić, 1999; Zakeri & Hirobe, 2010; Zielinski et al., 2009).
Chromosomal deviation due to complicated chromosomal rearrangements can be caused by genetic damage (Paz-Y-Miño et al., 1995), including aneuploidy (Thierens et al., 2000) and micronucleus formation (Doyle & Pariza, 1994; Sies & Stahl, 1995). A previous study estimated chromosomal deterioration in 37 hospital staff peripheral lymphocytes exposed to low IR. The micronucleus (MN) assay was utilized to evaluate the mutagens and radiation's clastogenic effect as a biological sign of genetic detriment. The results demonstrated that the level of MN in exposed workers was higher than in control groups (Maffei et al., 2002). Another study indicated that people working in nuclear medicine departments and diagnostic X-rays were exposed to IR and found to have chromosomal abnormalities in their peripheral lymphocytes; It was found that A four-fold elevation in the degree of chromosomal aberrations between the control and exposed groups without quantitative or arbitrary cytogenetic alterations between nuclear medicine and x-ray exposed personnel (Ballardin et al., 2007). They also conclusively showed that chromosomal aberration was higher in exposed technicians than in other professional groups (Kašuba, Rozgaj, & Jazbec, 2008). Another study established the low-level ionizing radiation's effects on 765 hospital staff's 48-hr peripheral lymphocytes vocationally exposed to IR that doubtful to stimulate chromosome abnormalities and comparing them to 200 control patients. The experimental groups demonstrated an elevation in all kinds of chromosomal aberrations (Trosko et al., 1997).
Cellular apoptosis
Physiological suicide is activated as a cellular response to a destructive agent is recognized as "apoptosis," also referred to as "planned cell death," a vital function in forming and Maturing a large variety of organs (Lobo, Patil, Phatak, & Chandra, 2010; Raff, 1992; Ushakova et al., 1999). According to studies, oxidative DNA damage induces apoptosis in murine T-cell hybridoma and ROS creation. Once upon a time, it has been postulated that oxidative stress is a frequent apoptosis mediator.
The ionizing radiations use as a positive medication in malignancy treatment, via inducing the apoptosis operation through a mechanism consisting of overproduction of ROSs in the cell the radiation-induced alteration in gene expression by ROS (Ushakova et al., 1999; Wolfe, Ross, & Cohen, 1994). Despite the many benefits of ionizing radiation, long-term exposure to IR will induce apoptosis in a healthy person as medical radiology workers have a hazardous effect on the cells. Ionizing radiation primarily causes hydroxyl and superoxide radical manufacturing (Lindahl, 1993; Ward, 1988). This means that when the antioxidant capacity of the enzymatic and non-enzymatic systems is insufficient, DNA is damaged (Ames, Shigenaga, & Hagen, 1993).
Figure 2
The radiation alters geneexpression and cell cycle checkpoints, resulting in damage to DNA that inducesapoptosis.
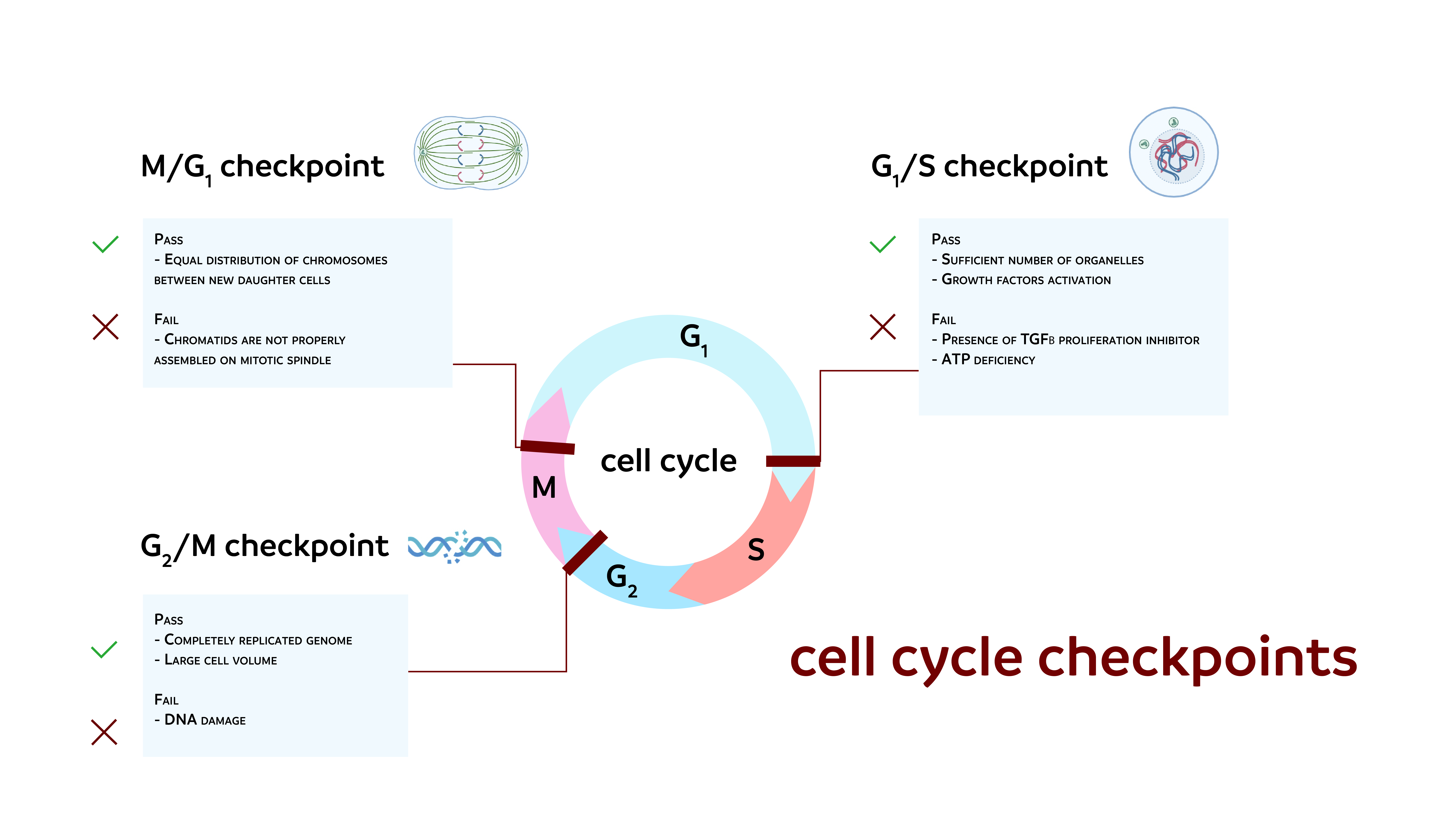
It is hypothesized that using antioxidants to scavenge ROS prevents apoptosis by lowering cell ROS levels, thus preserving membrane integrity (Salganik, 2001). Many proteins and factors may increase or decrease the apoptosis operation as Anti-APO-1 antibodies activate apoptosis in many tumors, and anti-Fas antibodies have a similar effect (Lobo et al., 2010). TNF, which has tumoricidal activity, is another element that promotes apoptosis (Westendorp et al., 1995); these are TRPM2 determine the second TRPM ion channel subfamily member. It has 1503-amino acid permeable Ca2+, Na2+, and K+ channels in humans (Hara et al., 2002; Miller, 2019; Nagamine et al., 1998). It can be present in various cells, such as those in the brain, hematopoietic system, and heart TRPM2 is involved in multiple physiological and pathological oxidative stress pathways (Miller & Cheung, 2016; Simon, Varela, & Cabello-Verrugio, 2013).
TRPM2 overexpression induced apoptosis, while TRPM2 reduction counteracted histone deacetylase inhibition-induced apoptosis (Huang et al., 2017; Miller, 2019; Orfanelli et al., 2015). TRP channels are frequently dysregulated in gastrointestinal tract cancers, resulting in alterations in cancer features such as increased invasion, migration, proliferation, and the inability to trigger apoptosis. Thus, TRP channels could be used as diagnostic markers (Stokłosa, Borgström, Kappel, & Peinelt, 2020). By suppressing apoptosis, cell survival can increase, as was suggested for the oncogene bcl-2. The bcl-2 structure as a family of proteins was discovered over 15 years ago, but its design and functional complexity are unknown (Siddiqui, Ahad, & Ahsan, 2015). Its gene product improved cell survival. It may preserve cells from apoptosis brought about with the aid of a variety of extra- and intracellular factors and plays a significant role in preventing apoptosis (Hockenbery, Nuñez, Milliman, Schreiber, & Korsmeyer, 1990; Niture & Jaiswal, 2012; Sentman, Shutter, Hockenbery, Kanagawa, & Korsmeyer, 1991; White, 1996). Bcl-2 overexpression can prevent apoptosis necrosis and protect ischemic tissue from oxidative stress caused by reperfusion (Kane et al., 1993; Saxena, Israels, & Israels, 2001; Zhao, Yenari, Cheng, Sapolsky, & Steinberg, 2003). Tumor-associated macrophages (TAMs) also enhance EC cells' epithelial-mesenchymal transition (EMT) and suppress their apoptosis (Wang, Ma, Li, & Su, 2022). The previous study had been published in Free Radical Biology and Medicine book ensures the dietary A mixture of antioxidants such as b-carotene, vitamin E, C, and rutin, besides zinc and selenium in fat-free curd granules, decrease the TRPM2 as a marker of apoptosis and increase the bcl-2 gene as a marker to prevent apoptosis after ionizing radiation exposure (Ushakova et al., 1999). Antioxidants, including Trolox, a water-soluble vitamin E derivative, inhibit apoptosis by lowering lipid membrane peroxidation and calcium uptake after irradiation (Mcclain, Kalinich, & Ramakrishnan, 1995).
Naturally occurring antioxidants
The antioxidant mechanism can be carried out by one of two methods: prevention or chain-breaking. When a free radical spreads to other molecules that form a radical chain, it breaks. A chain-breaking antioxidant helps to keep this chain stable. Lipid peroxidation is a well-known example of a chain reaction. Superoxide dismutase, glutathione peroxidase, and catalase are enzymatic antioxidants that may help avoid oxidation by steadying conversion metal radicals like copper and iron. or by slowing chain formation, for instance, by scavenging initiating free radicals (Pham-Huy, He, & Pham-Huy, 2008).
Two types of antioxidants are produced spontaneously in the body (endogenous antioxidants), which are further subdivided into enzymatic and non-enzymatic antioxidants, as well as externally provided antioxidants (exogenous antioxidants) (Anuj et al., 2016). Nutrient antioxidants belong to exogenous antioxidants (Sentman et al., 1991). Studies show that an antioxidant-rich diet has a significant long-term health benefit (Sin, Liu, & Lam, 2013; Willis, Shukitt-Hale, & Joseph, 2009). It is a well-known fact that many natural antioxidants, such as vitamin C be obtained from citrus fruits (oranges, lemons, etc.). Broccoli flowers, Blueberries, grapes, strawberries, red beans, prunes, kale, spinach, plums, alfalfa sprouts have been demonstrated to contain a high concentration of antioxidants (Cao, Russell, Lischner, & Prior, 1998; Grossman, Reznik, Tamari, & Albeck, 1994). These dietary antioxidants prevent or delay cell damage primarily through their capability to scavenge free radicals (Halliwell, 1995). These radioprotective agents can be administered before or after radiation exposure to diminish the consequences of IR-induced cell damage (Dowlath et al., 2021).
Effect of using antioxidants to reduce chromosomal damage
The severity of the DNA injury can be adjusted by curing cultured cells with antioxidants (Loft et al., 1996). According to the FDA, antioxidants are materials used to protect food by slowing retro-gradation discoloration due to oxidation (Bagchi & Puri, 1998; Bradley & Min, 1992; Kumar, 2011). The radioprotective properties of vitamins C, E, and carotene against radiation-induced chromosomal abnormalities are controversial. So, the antioxidant vitamins considerably reduced the chromosomal destruction in the cells subjected to radiation and defended against any future mischievous effects of IR possibly related to medical imaging (Mettler et al., 2011). A previous study reported that a blend of antioxidants was revealed to decrease double-strand DNA breaks formation (DSBs) in human cells in vitro with a dosage of 10 mGy of radiation (Kuefner et al., 2012).
Another study estimated that vitamins C and E decrease chromosomal abnormalities, mutations, and programmed cell death in cells. Also, vitamin A and N-acetylcysteine have been proposed to be efficacious in contrast to carcinogenesis caused by radiation (Sminia, Kracht, Frederiks, & Jansen, 1996). The effect of radiation protection has been demonstrated in animal models by administering antioxidants like superoxide dismutase (SOD) (Petkau, Chelack, Pleskach, Meeker, & Brady, 1975) and catalase (A & M, 1993; Galeotti, Borrello, & Masotti, 1990). Also, Flavonoids, associated with almost all plant parts, are widely accepted antioxidants that trap free radicals and block radical chain reactions (Zheng, Deng, & Zhang, 2022).
Vitamin E
Its essential antioxidant, neuroprotective and anti-inflammatory characteristics have established health benefits (Nesaretnam, 2008). It has also been demonstrated to modulate radiation-induced oxidative damage (Shin, 2003). It was reported that protocols or variations could provide the highest level of ionizing injury prevention, with dose reduction factors (DRF) ranging from 1.06 to 1.23 (Singh, 2014). Of these, α-tocopherol was mainly concentrated on, Because it has the maximum bioavailability, your body rapidly absorbs and metabolizes that form to its preference (Brigelius-Flohe & Traber, 1999).
The primary lipid-soluble antioxidant is α-tocopherol, which prevents the oxidation of membranes by interacting with lipid radicals during the lipid peroxidation chain (Traber & Atkinson, 2007). In addition, α-tocopherol induces the expression of other necessary radiation protection factors, which could be more effective than α-tocopherol itself. Various tocopherol analogs then showed the ability to induce cytokines and growth factors to mediate the effectiveness of radioprotection (Singh & Krishnan, 2015). In previous studies, subcutaneous use (100 IU/kg body weight) for α-tocopherol, 1 hour before and 15 minutes following β-irradiation, significantly increased mice survival for 30 days, DRFs of 1,06 (1 hour before) and 1,11. (15 min after) (Srinivasan & Weiss, 1992).
Alpha-tocopherol succinate
The α-tocopherol hemisuccinate ester derived from 𝛼-tocopherol has greater efficacy than α-tocopherol (Prasad, Kumar, Yan, Hanson, & Cole, 2003). In radiated mice, antioxidant enzyme expression was demonstrated to control and inhibit oncogene expression (Singh, Parekh, Brown, Kao, & Mog, 2011). TS has also been administered subcutaneously in mice 24 hours before irradiation at a 400 mg/kg dosage, which is compatible with recently published results (Singh et al., 2012; Singh et al., 2011). To be efficient radiation protectors, tocotrienols have been shown in animal models of acute radiation syndrome caused by whole-body exposure to 𝛾- or X-rays (Li et al., 2013; Li et al., 2015). Tocotrienol may be superior to antioxidant effectiveness due to its unsaturated aliphatic tail that helps tissue penetration (Singh et al., 2015). A single study demonstrated that tocotrienols had 1600 times the activity of α-tocopherol (Serbinova & Packer, 1994). Studies (Ghosh et al., 2009; Kulkarni et al., 2010) have shown that 𝛾 -tocotrienol (GT3) shields against radiation damage by raising hematopoietic progenitors, neutrophils, platelets, reticulocytes, and white blood cells. As demonstrated above, Subcutaneous administration at 24 h before exposure to all types of vitamin E analogs showed ARS safety effects (Ghosh et al., 2009; Sree, Srinivasan, Toles, Jobe, & Seed, 2002). Ascorbic acid, also known as "vitamin C," is a mono-saccharide antioxidant present in plants and animals (Lobo et al., 2010). Incubation of vitamin C at the recommended concentrations of WHO 1 h before ten mGy β irradiation was significantly reduced by 25 percent (p<0,0001) for dual strand breakings in peripheral blood lymphocytes (Brand et al., 2015).
Carotenoids
Lycopene and beta-carotene, for instance, are abundant in vegetables like tomatoes, carrots, and orange/yellow fruits (Nakamura et al., 2017), in addition to tomatoes and tomato products. Lycopene is the primary carotenoid (Islamian & Mehrali, 2015). Studies show that consuming tomato products increases the antioxidant's ability, lowering the risk of developing oxidative stress diseases (Burney, Comstock, & Morris, 1989; Sandhir, Mehrotra, & Kamboj, 2010). Furthermore, it has been suggested that LYC can protect against ionizing radiation-induced mutations (Çavuşoğlu & Yalçın, 2009). They estimated the radioprotective impact of lycopene on γ radiation-induced toxicity. In a study performed by (Forssberg, Lingen, Ernster, & Lindberg, 1959), the use of lycopene in both before and after x-ray dosage was found to be moderately curative when injected intra-peritoneally in irradiated mice as particulate suspension. In addition, many studies have indicated that a diet rich in lycopene and lycopene supplements protect against ROS products (Böhm, Edge, Burke, & Truscott, 2001; Sahin et al., 2006). Furthermore, it has been demonstrated that consuming 60 g of tomatoes purée daily improves lymphocyte DNA resistance to oxidative stress in 3 weeks and increases the concentrations of lycopene and ß carotene plasma (Riso & Porrini, 1997). Another study shows that 2.5 mg/kg of orally administered beta-carotene has been found to reduce chromosome damage followed by radiation to mice as measured with polychromatic micronucleate (Abraham, Sarma, & Kesavan, 1993).
Flavonoids
They are found naturally polyphenolic compounds with high antioxidant activities (Otto, 2010), the bulk of which are yellow phytochemicals extracted through flown—initially identified as radiosensitizing compounds (Hillman et al., 2001; Raffoul, Sarkar, & Hillman, 2007; Watanabe, Hirayama, & Kubota, 2007). Numerous studies have revealed radioprotective behaviors through free radical scavengers like hydroxyl radical (Kandaswami & Middleton, 1994). Devi and colleagues' research has discovered how a flavonoid derived through low-dose Ocimum preserves mice towards 2.0 Gy from γ -radiation of the whole body (Devi, Bisht, & Vinitha, 1998). One other in vivo research via Jagetia and his colleagues reported which bioflavonoid of grapefruit, naringin, safeguards against chromosomal harm caused by radiation (Jagetia, Venkatesha, & Reddy, 2003). One, even more, Benkovic and his colleagues documented that Alkaline comet assay was utilized to test the radioprotective impacts of propolis and associated flavonoids over whole-body irradiated rats (Benković et al., 2008).
Some flavonoids like orientin, quercetin, genistein, and even routine have been assessed in vivo as radioprotector (Hosseinimehr, 2007). Flavonoids have a c6–c3–c6 structure, with dietary significance subgroups (Rodriguez-Mateos et al., 2014), found all over the plant kingdom (Article, Banjarnahor, & Artanti, 2014). Then they seem to be a necessary component of our daily diet like fruitiness, vegetables, tea, nuts, seeds, stems, flowers, and wine (Article et al., 2014). In particular epigallocatechin-3-gallate (EGCG), soy isoflavones (such as daidzein, glycitein, and genistein) and silymarin from Silybum marianum, green tea epicatechin, baicalein of Scutellaria baicalein, pea apigenin either garlic apigenin, chrysin, isolated of propolis and quercetin from the onions outer skin have shown some protective influences against radiation cased damage (Otto, 2010).
In comparison to the control group, the critical flavonoids of baicalein, Scutellaria baicalein, used Chinese herbs in medicine, could limit the harm to DNA in cells of the blood of human following γ-irradiation. When contrasted to the control group, four Gy γ-irradiation of eight weeks old Swiss albino rats pre-treated with baicalein caused decreased DNA damage in bone marrow and cells of the blood whole body. That influence could be because of the alkyl also hydroxyl activity of baicalein radical scavenger (Gandhi, 2013). Chrysin (5,7-dihydroxyflavone) is a flavonoid that originates in honey, propolis, and many flora, particularly Passiflora caerulea. Its capacity to pass the blood-brain barrier (BBB) demonstrated empowerment. Its antioxidant impact also boosts levels in caspase-3 activity & malondialdehyde (MDA) throughout the male Wister rats' brains following a five Gy full-body irradiation (Mansour, Moawed, & Elmarkaby, 2017)
Melatonin(N-acetyl-5methyloxytryptamine)
A pineal gland hormone key regulator, including its axis of the neuroendocrine glands, is a potent free-radical scavenger and antioxidant (Pieri, Marra, Moroni, Recchioni, & Marcheselli, 1994; Pierrefiche & Laborit, 1995; Reiter, 1995). Indirect antioxidants and a direct scavenger of free radicals that stimulate antioxidant enzymes have been identified (Mallo et al., 1990; Shirazi, Mihandoost, Mohseni, Ghazi-Khansari, & Mahdavi, 2013; Tan et al., 1993), for example, GSH-Px, SOD, glutathione reductase, and catalase (Karbownik & Reiter, 2000). It also raises GSH intracellularly by promoting the creation of an enzyme that limits the rate, γ-glutamylcysteine synthases, that prevents lipoxygenase and the per-oxidative enzymes nitric oxide synthase (Shirazi et al., 2010). In addition, our current database, according to another research, demonstrated that radiation exposure increased MDA and decreased GSH standards within mice's lenses. However, those values were in natural borders when taking melatonin (Shirazi et al., 2013).
Melatonin has been documented to decrease the 8-hydroxy-2 - deoxyguanosine composition. The weakened product of DNA happened due to radicals, sixty towards seventy times greater efficiency than most other classical antioxidants (tocopherol or ascorbate) (Qi et al., 2000). Consequently, melatonin serves as a direct free radicals scavenger and indirectly activity activator in antioxidant protection systems (Cagnoli, Atabay, Kharlamova, & Manev, 1995; Qi et al., 2000; Tan et al., 2002) In another research investigated essential melatonin function for decreasing apoptosis which is induced by eradiation in rats cervical spinal cord by (Shirazi et al., 2010). The findings of this research point out the melatonin had protectively impacted towards eradiation-caused apoptosis. Melatonin raised Bcl-2 gene expression substantially to reduce Bax gene expression within the irradiated spinal cord, which is crucial in that research. The melatonin's function as a substance inhibits cells from dying throughout normal cells of recent areas for investigation. Knowledge remains limited also. In another study done by Fernandez-Gil et al., melatonin may prevent intestinal obstruction lumen cells from harmful chemicals produced during oral mucosa radiation treatment. They utilized Wistar-Adult male rats administered three % melatonin gel (total melatonin dose was 45 mg/day for 21 days). When compared to the irradiated but non-melatonin-treated group, there was a significant rise in the activity and protein levels of GPx, GR, SOD2 and a significant decline in the activation of inflammasome in the small intestine in the melatonin-treated group (Nuszkiewicz, Woźniak, & Szewczyk-Golec, 2020).
Combinations of dietary antioxidants
Various sources may also produce synergistic antioxidant effects (Makanjuola, Enujiugha, Omoba, & Sanni, 2015). Fruit extracts have been demonstrated to have high antioxidant and anti-proliferative properties; thus, the phytochemical mixture found in fruits and vegetables is critical for significant antioxidant and anti-cancer action (Liu, 2003). In addition, various in vivo and in vitro studies show that giving antioxidants in combinations leads to increased radioresistance in normal tissue than when given individually (Prasad, 2004; Prasad, 2005). For instance, consider the mixture of vitamin E and vitamin C, which provides adequate membrane protection and better radioresistance in cells (Prasad, 2004). Irradiated mice injected with SOD or, more efficiently, the combination of catalase and Trolox suggested a decline in lipid peroxides and apoptotic markers (Hernández-Flores et al., 2005), that consider a water-soluble analog of the free radical scavenger α-tocopherol (Hamad, Pekmez, Karaer, Temizkan, & G, 2010).
Also, it is good to mention that Satoh et al. asserted that Trolox's antioxidant capacity outperforms that of α-tocopherol. In vitro, both selenium and vitamin E and their mixture have been shown to attenuate radiation-induced changes. Negre-Salvayre et al. used a mix of a-tocopherol, ascorbic acid, and the quercetin glycoside, rutin, to protect lymphoid cell lines from per-oxidative stress caused by oxidized LDL. These researchers also discovered that flavonoids could prevent the cytotoxicity of oxidized LDL in two methods: by decreasing the lipid peroxidation of LDL (caused by UV irradiation) or by obstructing the cytotoxicity of previously oxidized LDL at the cellular level. The extracts produced from tea and ginger mixes exhibited more robust synergistic and additive benefits and less adverse synergistic effects. As a result, it is proposed that the mixture of phytochemicals from various sources be encouraged to maximize the benefits in health received from them (Makanjuola et al., 2015). A study done by M Gorenberg involved 16 consecutive patients divided into two groups: six patients got the antioxidant combination (1-Curcumin Yerba mate, 2-Gingko Biloba, 3-N' acetyl cysteine, 4-Astaxanthin (AST), 5-Vitamin D (VD), 6-Quercetin, 7-D-alpha-tocopherol (VE), 8-Ascorbic acid, 9-Epigallocatechin-3-gallate) and ten patients served as controls. The paired t-test demonstrated a significant difference between the patient's groups treated with an antioxidant cocktail and the untreated patients' control group, p<0.005 (Gorenberg et al., 2021).
The risks capabilities of excess taken of dietary antioxidants
The excessive intake of supplementary antioxidants will get the opposite result. Previous research has found that antioxidant use is harmful. Vitamin E higher doses, for example, have been proven to increase all-cause mortality in patients (Miller et al., 2005). Supplementation with remote forms of vitamin C, vitamin E, and carotene at high doses boosted oxidative destruction caused by lymphocytic DNA (Podmore et al., 1998). Green tea's dietary antioxidant, on the other hand, exhibits cytotoxicity at more significant concentrations while showing resilience to oxidative stress at low dosages (Galati, Lin, Sultan, & O'brien, 2006). Overuse of antioxidants like vitamin E, vitamin C, and N-acetyl cysteine, for example, results in reductive stress, which is equally as damaging to cells as oxidative stress and has been related to diseases such as cancer and cardiomyopathy (Henkel, Sandhu, & Agarwal, 2019). In addition, an inappropriate intake of high doses of antioxidants may put the female organism's redox balance into reductive stress, which can severely impact fertilization and embryo development (Miller et al., 2005). Recent large-scale international cohort studies suggest an increased risk of cancer due to low-dose chronic exposure (Leuraud et al., 2015; Richardson et al., 2015).
New standards protocol followed by the medical, occupational radiation staff; Radiation protection is critical for medical staff to ensure that the potential risks do not exceed the advantages of diagnostic imaging (Zervides et al., 2019). International standards and recommendations, such as those established by ICRP and the International Atomic Energy Agency (IAEA) Basic Safety Standards, serve as the foundation for radiation safety legislation and guidelines (Part, 2011; Valentin, 2007). ICRP developed a radiation protection policy that has been adopted by the majority of developed countries worldwide (ICRP, 1991).
The concept of dose limits is one of the framework's guiding principles. The ambient radiation exposure dose must not exceed the dose limit given in millisieverts (mSv) per year. The radiological personnel limit is 20 mSv/year, and special attention is given to the lens dose according to ICRP publication "Statement on tissue reactions recommendation in 2011" (ICRP, 2011). In addition, the use of optimization approaches has decreased radiation exposure of both patients and interventional personnel (Pekkarinen, Siiskonen, Lehtinen, Savolainen, & Kortesniemi, 2019). Although these international projects were able to collect empirical proof of health impacts in occupationally exposed people and produced more reliable estimates of dose-response than single-nation research, results from these researches at low dosage ranges, particularly less than 100 mSv, should be regarded with carefulness due to broad confidence intervals for exposure assessment and inadequate information on confounders. In addition, given that baseline dangers vary from country to country, it is important to generalize the results to other populations with caution (Seo et al., 2018).
As a result, national studies are mandatory to assess the health impacts of low-dose ionizing radiation that reflect and represent the country's distinctive characteristics, as well as precise information on confounding factors (Seo et al., 2018). Recently, the recognition of referring physicians' lack of knowledge about radiation exposures encountered during diagnostic radiological treatments has grown (Azmoonfar et al., 2016). In general, various studies have already revealed medical professionals' lack of knowledge and awareness concerning their comprehension of ionizing radiation or the use of procedure-related equipment (Yurt, Çavuşoğlu, & Günay, 2014; Zhou, Wong, Nguyen, & Mendelson, 2010). Overall, radiation personnel needs to have a clear history of ionizing radiation and its adverse effects.
Conclusion
Last decades, many researchers' efforts using multiple resources have been trying to figure out promising approaches to protect the medical radiology staff from ionizing radiation hazards. Meanwhile, the new trend of therapies now focuses on natural products strategies that may protect us from long-term ionizing radiation. Dietary antioxidants and natural supplements as a prophylactic tool consider as one of the most common prevention strategies against IR. Moreover, most of the available studies recommend a regular natural diet containing a satisfying amount of dietary antioxidants during the daily work of radiologists, technologists, and radiology nurses. However, still, there is a need for more research and studies to cover the gaps and minimize the risks of Oxidative stress and long-term exposure to ionizing radiation.