Introduction
The challenging task of the 21st-50th century is to clean the environment in an eco-friendly and sustainable way, proper resource utilization, efficient use of nutrients, diseases control and enough availability of food (Kumar, Tokas, Kumar, & Lal, 2018). These tasks can be fulfilled by employing nanotechnology in these fields (Wiyaratn, Appamana, Charojrochkul, Kaewkuekool, & Assabumrungrat, 2012). Nanotechnology is a branch of science dealing with studies involving Nano size (10-9 size of meter) particles (Mandal, Bolander, Mukhopadhyay, Sarkar, & Mukherjee, 2006). Nano can be applied to any unit of measure for example you can consider Nano-grams and Nano-liters. The concept of nanoscience was first time driven by Richard Feynman in 1959 and from then it was growing very fast in all areas of research and development worldwide (Jain, Kachhwaha, Jain, Srivastava, & Kothari, 2010). Nowadays, nanoscience is the subject of extensive research in all areas (Li et al., 2007). It may bring the next industrial as well as the green revolution in the world (Sathishkumar et al., 2009). Nanoscience provides many environmental benefits to agriculture. These may be in the form of Nano-fertilizer, disease detectors, soil fertility enhancers, Nano-sensors and reduction of pollutants like heavy metals in soil. The specific nanoscience application for onsite remediation of soil, air, groundwater and wastewater treatment are also in development. The size of nanoparticles has significance in their physiological and biochemical properties. It makes it behave completely different from its main part. The reduction in the size changes redox properties, thermodynamic properties and internal cohesive forces of the particle that causes easy delivery and interaction of the particle with the active site (Li et al., 2007). Nanomaterials may be organic, inorganic, biogenic or natural based on their process of formation. Nanoparticle synthesis using microorganisms or plants comes under biogenic or green nanoscience technology. Microbes are the emerging Nano-factories and have potentials for environmental clean-up methods. It may provide a better alternative than inorganic nanomaterials for diseases control in plants (Hsu, Lou, Chou, Hsu, & Han, 2015). Nanoscience is a persistent solution for our environment. Although it is a new area still it is growing rapidly.
Nanoscience can help us to design new materials with peculiar properties and reproducibility for agricultural development (Ndlovu, Mayaya, Muitire, & Munyengwa, 2020). In this concern, the scientific community should continuously focus on a very challenging and relevant research’s direction, which is the development of nanoparticles capable of heavy metal remediation from soil and water. The concept of nanoscience may help us to understand the mechanism of transformation of heavy metals into plants and animals, more accurately at the molecular level. The synthesis of materials at the Nano-size dimension will facilitate us to design new useful systems for heavy metal removal from plants. Although nanoscience is a vast field, this review has been designed to be focused on the synthesis, types, current research development in the areas of biosensors for agriculture and their safety precautions to revolutionize the agricultural sector by ensuring sustainable food security for coming generations across the globe.
Nanoparticles, their types, nature, sources and importance in plant production and growth
The particle of very small size (<100 nm), of any material, is called a nanoparticle of that particular element. They are atomic or molecular aggregates of 1 – 100 nm dimension and their physicochemical properties modify drastically as compared to the bulk material (Table 1). The properties of nanoparticles depend and may vary with their size i.e. the size of nanoparticles define their properties. Nanoparticles are more mobile and more reactive. They are roughly divided into organic and inorganic. The first category of organic nanoparticles comprises carbon nanoparticles. The second category inorganic nanomaterials comprise magnetic nanomaterials, noble metal nanoparticles like silver, gold nanoparticle and semiconductor nanoparticles like titanium dioxide, zinc oxide. Nanomaterials are also produced naturally by volcanic and lunar eruptions (Li et al., 2013). These are known as natural nanoparticles but there are other classes of nanoparticles that are produced by anthropogenic activities like the exhaust of diesel, coal combustion and fumes of welding works; these are known as incidental nanoparticles. The nanoparticles synthesized by man are called engineered nanoparticles. The man-made or engineered nanoparticles includes nano zinc, nano aluminium, TiO2, ZnO, quantum dots etc. (Hočevar et al., 2013). The smaller size of nanoparticles makes them more useful for use as sensors that can be easily deployed in remote locations. Currently, nanomaterials are being proved successful both in efficiency as well as cost-effective and also in the environmental friendly way in utilizing them as an alternative for current treatment materials for agricultural remediation (Rao, Lu, & Su, 2007; Rodríguez-León et al., 2013). The use of biologically synthesized nanomaterials has been increasing in the field of agriculture because of their stable, eco-friendly and cost-effective nature (Popescu, Alin, & Lőrinczi, 2010). They should be utilized in wider applications. The biological synthesis of nanomaterials is more advantageous over other methods due to ease of fast synthesis, ease of toxicity control, ease of size control, cost-effective and environmentally friendly methodology (Umashankari, Inbakandan, Ajithkumar, & Balasubramanian, 2012). Nanoscience is being utilized extensively for the protection of agriculture from bacterial disease and pollutants including chemicals like heavy metals (Petla, Vivekanandhan, Misra, Mohanty, & Satyanarayana, 2011). It includes a way to encapsulate agrochemicals and slowdown their release in plant protection. Some examples of nanoparticles used in controlling seed germination, microbial and fungal attacks in increasing agricultural production are summarized in Table 2.
Table 1
Some examples of the material changing nature of their nanoparticles compared with their bulked forms.
Table 2
Some examples of nanoparticles used in controlling seed germination, microbial and fungal attacks.
Sl. No. | Contribution of Nanoparticles |
1. | Silver, gold, oxides of titanium and zinc Nanomaterials (NM) can be used in some plants species for positive effects on germination and growth. |
2. | The carbon nanotubes can be used as regulators of seed germination and plant growth. |
3. | Multiwall carbon nanotubes (MWCNTs) can enhance the growth of tobacco cell culture by 55 - 64% when compared to control at a wide range of concentrations from 5 - 500 µg ml-1 |
4. | At low concentrations, activated carbon enhanced cell growth |
5. | Nanotechnology provides controlled and efficient use of fertilizers, pesticides and other agrochemicals for better growth and production of plants (Gudkov et al., 2020). |
6. | Chitosan nanoparticles are proved more effective antifungal agents against the pathogen (Fusarium solani). |
7. | Silver nanoparticles act as antimicrobial agents and protect plants from microbial diseases. |
8. | Copper nanoparticles may act as bio-pesticides and protect plants from pests. |
9. | Zinc based nanoparticles inhibit fungal growth by damaging conidiophores. |
Synthesis of Nanoparticles
Traditionally, there are several methods for generating nanoparticles (Figure 1) including abrasion, ion embedding, pyrolysis, lithography and milling. In abrasion, macro- or micro-scale elements are crushed in a ball mill, an earthly ball mill, or other size-reducing apparatus. The resulting particles are air categorized to recover nanoparticles. In pyrolysis, a vaporous precursor (liquid or gas) is forced through an orifice at high pressure and burned. The resulting solid (a version of soot) is air categorized to recover oxide particles from by-product gases. Old-style pyrolysis often results in aggregates and agglomerates rather than single primary particles. Ultrasonic nozzle spray pyrolysis (USP) on the other hand aids in preventing agglomerates from forming. It is called the top-down approach in nanomaterial synthesis. The other one is the bottom-up approach which includes gas condensation, physical vapour deposition, chemical vapour deposition, laser ablation, molecular beam spraying, spraying, aerosol, micro-emulsions, thermal decomposition, chemical precipitation, solvothermal synthesis, sol-gel method, photochemical, sonochemical, chemical reduction, electrochemical reduction and hydrothermal production. These methods are costly for nanoparticle synthesis, there are other methods to produce nanoparticles cheaply as:
Biogenic production of various nanoparticles
The microorganisms and plant extracts are used generally for a cheaper synthesis of nanoparticles. The main reaction involved in the biosynthesis of the nanoparticle is the oxidation/reduction reaction. It is a bottom-up approach for the synthesis of the nanoparticle. The biochemical antioxidants in the plant sample and biochemical enzymes in the microbial sample are responsible for the reduction of metal into their nanoparticles. Currently, there are millions of tons of production of nanoparticles worldwide and is probable to increase intensely in the near upcoming (Sekhon, 2010). The nanomaterials are materials having a particular dimension between 1 – 100 nm, so along with vascular plants; the microorganisms like bacteria, yeasts, algae, fungi, and actinomycetes may be used for nanoparticles biosynthesis (Saxena, Sharma, Gupta, & Singh, 2014).
Plants utilized in Nanoparticle synthesis
Nanoparticle synthesis from plant extract is called a green synthesis of the nanoparticle (Shebl et al., 2019, 2020). Currently, it is gaining prominence because of the single-step involved in biosynthesis. So, it is a time-saving process along with no toxicants and the presence of natural capping agents (Saxena et al., 2014). Plants material are easily available, they are safe to handle and possess a large variability of metabolites that aid in reduction. All these reasons make plants more advantageous over others for nanoparticle synthesis.
Figure 1
The general outline of various methods involvedin the synthesis of nanoparticles. Dynamic light scattering (DLS) and X-ray diffraction (XRD) is used for the characterization of Nano-powders of any size.
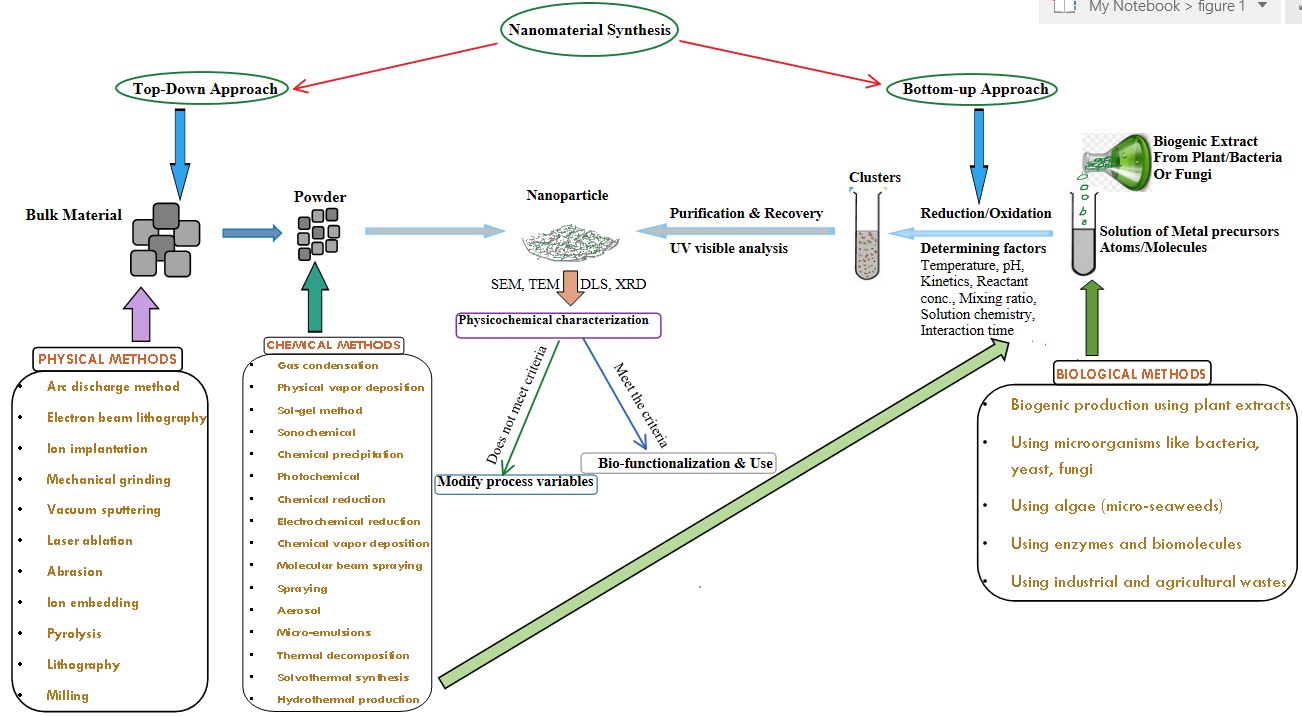
Currently, there are many plants are being investigated for their importance in nanoparticles synthesis (Table 3). Phytochemicals consume less time for the reduction of metal ions, compared to fungi and bacteria. It shows plants materials as a better candidate for the biosynthesis of nanomaterials, compared to bacteria and fungi. By utilizing plant tissue culture and downstream processing techniques, one can synthesis nanomaterials of metallic and oxide at an industrial scale if the metabolic status of the plants are addressed appropriately. It is found that the nature of nanoparticles produced from this method varies with the plant to plant, mode of application, size, and quantity (Mukherjee, 2010). It is revealed by the scientist that the investigation on nanoscience basically for plants is in its early stages; more depth study is required to reveal about biochemical, physiological and molecular mechanisms of plants in concern to nanomaterials and more work is required to explore the mechanism of nanomaterials actions, their behaviour towards biomolecules and their effect on gene regulation and expressions in plants. Generally, plant-based nanoparticles involve three mechanisms viz. adsorption, reduction and desorption mechanisms in the heavy metal removal process from plants, soil and water (Figure 2).
Figure 2
General mechanisms involved in heavy metal removal by plant-based nanoparticles from plants, soil and water. An example for the removal of Cr(VI) by Fe3O4@Zr-CTS microsphere is illustrated here (Chen et al., 2017).
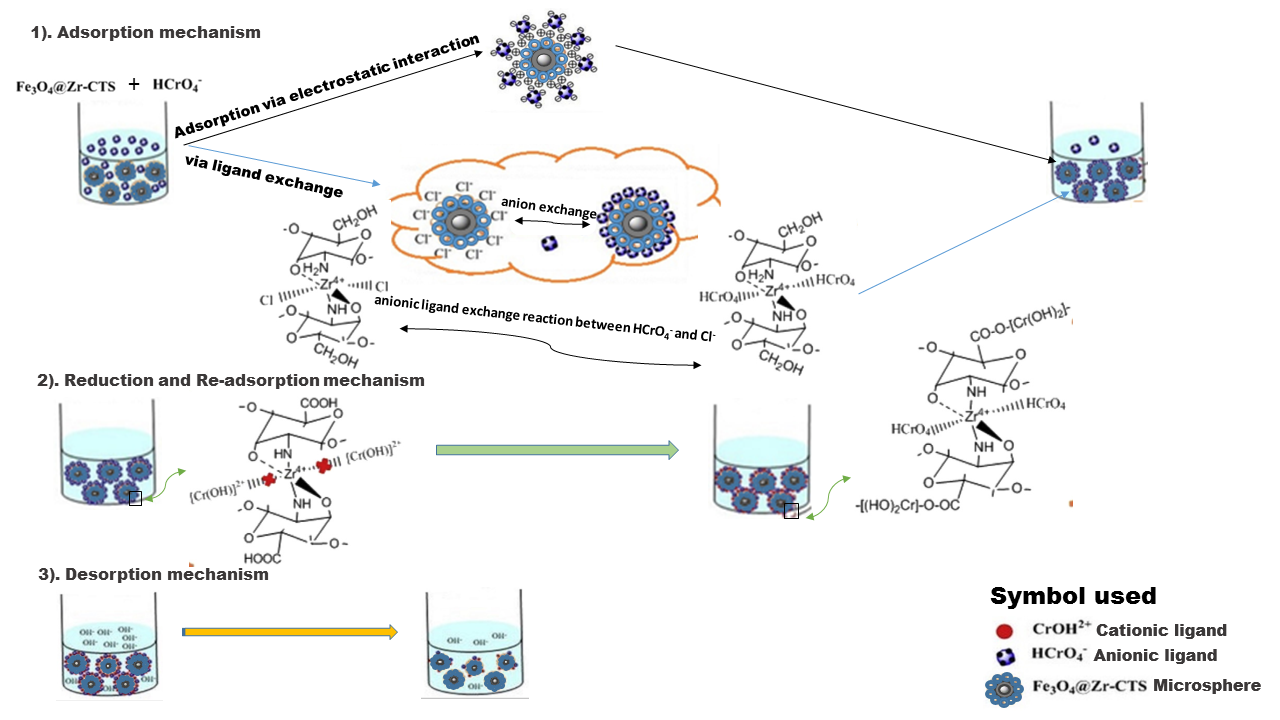
Table 3
Plants used for the synthesis of nanoparticles.
Nanoparticles | Plants species | Purpose | References |
Gold and silver nanoparticles | Citrus sinensis Diospyros kaki (Persimmon) Pelargonium graveolens Hibiscus rosa sinensis Coriandrum sativum Emblica officinalis Phyllanthium Mushroom extract | Contaminant to be removed Chlorpyrifos and Malathion | (Siddiqui, Al-Whaibi, Firoz, & Al-Khaishany, 2015) (Sathyavathi, Krishna, Rao, Saritha, & Rao, 2010) (Parida, Bindhani, & Nayak, 2011) (Reddy, Murthy, Srilakshmi, Rao, & Pullaiah, 2015) (Ankamwar, Chaudhary, & Sastry, 2005) (Kavitha et al., 2013) (Philip, 2009) |
Gold nanoparticles | Terminalia catappa Banana peel Mucuna pruriens Cinnamomum zeylanicum Medicago sativa Magnolia kobus and Dyopiros kaki Allium cepa L. Azadirachta indica A. Juss. Camellia sinensis L. Chenopodium album L. Justicia gendarussa L. Macrotyloma uniflorum (Lam) Verde Mentha piperita L. Mirabilis jalapa L. Syzygium aromaticum (L) Terminalia catappa L. Amaranthus spinosus | Contaminant to be Removed Inorganic-mercury, Used in biosensors | (Ankamwar, 2010) (Bankar, Joshi, Kumar, & Zinjarde, 2010) (Subramanian, 2010) (Blaney, Cinar, & Sengupta, 2007) (Kathad & Gajera, 2014) (Pavani, Swati, Snehika, Sravya, & Silrisha, 2012) (Parashar, Parashar, & Sharma, 2009) (Boruah, Boruah, Sarma, Medhi, & Kumar, 2012) (Dwivedi & Gopal, 2011) (Fazaludeen, Manickam, Ashankyty, Ahmed, & Beg, 2012) (Aromal, Vidhu, & Philip, 2012) (Mubarakali, Thajuddin, Jeganathan, & Gunasekaran, 2011) (Vankar & Bajpai, 2010) (Raghunandan et al., 2010) |
Silicon-Germanium (Si-Ge) nanoparticles | Freshwater diatom Stauroneis sp. | Used in hydroponics-form, soil supplementation or foliar spraying for plant growth and development | |
Silver nanoparticles | Elettaria cardamomom Parthenium hysterophorus Ocimum sp. Euphorbia hirta, Nerium indicum Azadirachta indica Brassica juncea Pongamia pinnata Clerodendrum inerme Gliricidia sepium Desmodium triflorum Opuntia ficus indica Coriandrum sativum Carica papaya (fruit) Pelargoneum graveolens Aloe vera extract Capsicum annum Avicennia marina Rhizophora mucronata Ceriops tagal Rumex hymenosepalus Pterocarpus santalinus Sonchus asper | Contaminant to be Removed Pathogenic bacteria E. coli, Staphylococcs aureus | (Gnanajobitha, Annadurai, & Kannan, 2012) (Parashar et al., 2009) (Koduru et al., 2011) (Priya, Selvi, & Paul, 2011) (Gericke & Pinches, 2006) (Raut, Kolekar, Lakkakula, Mendhulkar, & Kashid, 2010) (Farooqui, Chauhan, Krishnamoorthy, & Shaik, 2010) (W. et al., 2009) (Ahmad et al., 2011) (Sathyavathi et al., 2010) (Jain, Daima, Kachhwala, & Kothari, 2009) (Chandran, Chaudhary, Pasricha, Ahmad, & Sastry, 2006) (Li et al., 2007) (Gnanadesigan et al., 2012) (Umashankari et al., 2012) (Dhas, Mukerjhee, & Chandrasekaran, 2013) (Rodríguez-León et al., 2013) |
Palladium nanoparticles | Cinnamomum zeylanicum Blume. Cinnamomum camphora L. Gardenia jasminoides Ellis. Soybean (Glycine Max) L. | Contaminant to be Removed Chlorinated methane | (Smitha, Philip, & Gopchandran, 2009) (Yang et al., 2010) (Petla et al., 2011) |
Magnetic Nanoparticles | Aloe vera | Heavy metal removal | |
Nanoparticles of silver, nickel, cobalt, zinc and copper | Brassica juncea, Medicago sativa and Helianthus annuus | Utilization in gas sensors, biosensors, cosmetics, drug-delivery systems and antimicrobial | |
Platinum nanoparticles | Diopyros kaki Ocimum sanctum L. | Cellular Toxicity, Apoptosis, and Genetic Damage |
Role of bacteria utilized in Nanoparticle synthesis
Bacteria possess the peculiar property of mobilization and immobilization of elements and in some cases, they may precipitate metals even in nanometer size. Therefore, Bacteria are called bio-factory for the production of nanomaterials like silver, gold, palladium, titanium, magnetite, cadmium and platinum. It is a new method of nanoparticle synthesis. In this method, bacterial enzymes are involved in catalyzing a specific breakdown reaction and producing nanoparticles (Bali, Razak, Lumb, & Harris, 2006). The polysaccharides, vitamins, enzymes, biodegradable polymers and biological systems may be utilized for the synthesis of nanoparticles in the same way. The enzymes which are produced in extracellular secretion possess more advantage of the production of a large number of nanomaterials ranging size between 100 – 200 nm in a comparably pure form, and free from other materials like cellular proteins. These nanomaterials may be further purified by filtration easily. The metal binding and S-layer properties of bacteria make them more useful in the technical application of bioremediation and nanoscience. The different types of bacteria used for nanomaterials are shown in Table 4. The mechanism involved in silver nanoparticle synthesis by Pseudomonas aeruginosa is explained in Figure 3. The quality of nanoparticles produced by these techniques may be controlled by optimization of bacterial growth controlling parameters like enzymatic activities and cellular activities. Thus, there is a lot more to study to make proper use of this technique for nanomaterial synthesis. It is a more appealing field because of an ecofriendly, more stable, safe and less expensive process.
Table 4
Bacteria are used for the synthesis of nanoparticles.
Nanoparticles | Bacterium species | Purpose | References |
ZnS nanoparticles | the family Desulfo bacteriaceae | Controls growth, germination rate and development of plants | |
CdS nanoparticles | Clostridicum thermoaceticum Klebsiella aerogens Escherichia coli | Controls soil health, Insect and microbial population in soil | |
As-S nanotubes | Shewanella sp. | Provide useful materials for novel nano- and opto-electronic devices | (Ahmed, Kalathil, Shi, Alharbi, & Wang, 2018; Jiang et al., 2009) |
Palladium nanoparticles | Desulfovibrio desulfuricans NCIMB 8307 | Remove cellular toxicity and damages | |
Gold nanoparticles | Alkalothermophilic actinomycete Thermomonospora sp. Pseudomonas aeruginosa Lactobacillus strain | Used in water remediation of Dichlorophenol Trichlorobenzene Chlorinated ethane | |
Magnetic nanoparticles | Magnetosirillium magneticum Sulphate reducing bacteria | Used in nucleic acid detection, genetic manipulation, diseases detection | |
Gold nanowires | Rhodopseudomonas capsulate | Used to cleanup oil, organic pollutants in water and soil sediments and might help improve irrigation water quality in agriculture | |
Silver nanoparticles | Bacillus cereus Oscillatory willei NTDMO1 Escherichia coli Pseudomonis stuzeri Bacillus subtilis Bacillus sp. Bacillus cereus Bacillus thuringiensis Lactobacillus strains Pseudomonas stutzeri Corynebacterium Staphylococcus aureus Ureibacillus thermosphaericus | Used to control pests via pesticides, root promoting and pathogen inhibitory, antifungal agent, Used in plant tissue culture techniques and grafting experiments | (Babu, Gunasekaran, & P, 2009) (Gurunathan et al., 2009) (Klaus-Joerger, Joerger, Olsson, Granqvist, & C.-G, 2001) (Husseiny, El-Aziz, Badr, & Mahmoud, 2007) |
Yeast and fungi in Nanoparticle synthesis
Fungi produce a larger amount of extracellular enzymes than bacteria, which shows more influence on nanomaterial synthesis. The documented mechanistic aspect governing nanoparticle synthesis by using fungi are explained here. The nanomaterials with well-defined dimensions can be synthesized by using fungi. Compared to bacteria, fungi can be used for larger production of nanomaterials because fungi secrete a larger amount of proteins which directly increases the formation of nanomaterials. Moreover, the protein isolates from fungi can also be used for nanoparticle formation. The enzymes produced by fungi during metal nanoparticle synthesis reduce salts to their metallic solid nanomaterials by the catalytic effect (Saxena et al., 2014). It is major weaknesses of this synthesis method by microorganisms and if need be corrected if this method is to participate with other methods. The other disadvantage of this method is that it generates nanomaterials at a slower rate than detected when plant extract is used. The other thing to be considered for the fungal synthesis of nanomaterials is that fungi should possess certain properties like high production of required metabolites/enzymes, high growth rate, easy handling in large scale production and require low cost for production techniques. Generally, fungi are observed as the best source for nanomaterial synthesis over other biological systems because of their easy handling, cost-effectiveness, and wide diversity. Genetic engineering may be employed for an ecofriendly and improved method of nanoparticle synthesis (Shankar, Ahmad, Pasricha, & Sastry, 2003). In terms of the quality of nanomaterials and utilization of nutrients for microbial growth, yeast strains have assured benefits over bacteria (Popescu et al., 2010).
Role of Nanoscience in detecting plant diseases
There is a strong need to detect plant diseases in the early stage so that they can be protected from microbial agents and a great loss of food and agriculture can be prevented. Plant diseases are the most limiting factor for reducing crop productivity; the initial requirement to achieve control of plant disease is to recognize their causal organisms correctly. The identification process by involving traditional methods consumes a lot of time and inputs. But nanotechnological techniques such as nanoparticles and quantum dots (QDs), nano-imaging, nanostructured platforms, and nanopore tools for DNA sequencing requires less time and can be used for enhancing crop production and to ensure high-quality monitoring. In recent years, nanotechnology has emerged as a new diagnostic tool. Nano diagnostic kits help in preventing any epidemics at a much early stage which quickly detect the potential of plant pathogens and allows experts to help the farmers, thus nanotechnology can be applied for more cost-effective, quick, and accurate diagnostic plans of plant diseases. Nanotechnology may also be used for the delivery of pesticides at targeted/infected plant tissues, for pathogen detection and control of wound healing in plants. Nanoscience can be utilized in real-time monitoring systems, distributed throughout the fields, to monitor soil conditions and crop conditions, by providing or connecting an autonomous nanosensor to a GPS. This technique reduces the time required for observation of the crop in fields. It provides results within a few hours that are more accurate, simple and portable. This technique does not involve any complicated procedures and is so simple to be utilized by the farmers. The union of nanoscience with sensors technology may create new methods which allow early detection of diseases development and environmental changes. Nanoscience provides a new way of changing crop management methods and improving plant nutrition. It reduces the problem of leaching, photolytic degradation, hydrolysis, and microbial degradation. It has reduced the wastage of nutrients by providing how a very low amount of required minimal effective concentration, reach the target site of crops.
Nanomaterials for heavy metals removal
Heavy metals are distributed among soil, air, and water by natural or human activities like weathering, biodegradation, volcanic eruptions and mining, agrochemical industries; respectively. Heavy metals are increasing with human civilization. Any element having an atomic weight greater than 63.5 and having a specific gravity greater than 5.0 can be called heavy metal (Mueller et al., 2012). Heavy metals are divided into toxic metals (Hg, Cr, Pb, Zn, Cu, Ni, Cd, Co, Sn); precious metals (Pd, Pt, Ag, Au, Ru) and radionuclides (U, Th, Ra, Am). These metals are non-biodegradable and very difficult to eliminate naturally from soil, air, and water. Generally, all metals are toxic when their values increase from their permissible limits. Heavy metals cause toxic effects in the human’s body when it exceeds the permissible limits (Lee, Shin, Jun, Lee, & Jang, 2014). Various technologies have been used for heavy metal remediation like precipitation, reverse osmosis, advanced oxidation, coagulation, photo-electrochemistry, and flocculation. Each differs in the degree of remediation efficiency (Turhan, Avcıbası, & Sahiner, 2013). Currently, nanoscience has provided a great approach for heavy metal toxicity remediation by providing different nanomaterials. This technique is very beneficial over other techniques, some of the benefits are increased efficiency, reduced consumption and substation of more abundant and less toxic materials than ones used before. Generally adsorbing nanomaterials have shown great and effective work against heavy metal toxicity removal (Zhong, Ma, Cao, Chen, & Xue, 2009). Nanoparticles for heavy metal removal are divided into carbon-based nanoparticles (carbon nanotubes and graphene) and inorganic nanoparticles (based on metal oxides and metals). Different combination of nanoparticles is also developed for heavy metal removal. The disposal of nanoparticles may lead to release in the aquatic ecosystem which may prove harmful for them, this should be solved. The plants utilized in heavy metal removal are summarized in Table 5. The interaction of nanoparticles with plant’s biomolecules and removal of heavy metal cations and anions is explained in Figure 4.
Carbon-based nanomaterials
Activated Carbon
Activated carbon is produced from freely available carbonaceous precursors such as wood, coal, coconut shells and agricultural wastes. They possess high porosity and a large surface area. They are broadly used in wastewater treatments. These nanomaterials possess a high potential for the removal of organic and inorganic pollutants from contaminated water. Activated carbon has a significant weak acidic ion-exchange character which is involved in the removal of trace metal contaminants from wastewater by adsorption. (Ewecharoen, Thiravetyan, Wendel, & Bertagnolli, 2009) in his work observed the adsorption of pentavalent arsenic on granular activated carbon. The activated carbon synthesized from coconut tree sawdust was observed as an adsorbent for Cr (VI) and removes from wastewater (Park & Kim, 2005). Activated carbon is investigated for adsorption and stability of mercury on it (Samuel, Selvarajan, Chidambaram, Patel, & Brindhadevi, 2021). The adsorption ability of powdered activated carbon was tested against Cu (II) and Pb (II) and was found 0.85, 0.89 µmol g-1; respectively. A new sodium polyacrylate grafted activated carbon was synthesized by gamma radiation to enhance functional groups on its surface. This procedure may be applied to other adsorbents to enhance the efficiency of metal ions adsorption by activated carbon. Because of the low cost and high adsorption ability of activated carbons, they are appropriate materials for heavy metal removal.
Carbon nanotubes
Carbon nanotubes are like pillars for nanoscience. They are generally used in nanomaterial synthesis. Carbon nanotubes are divided into two groups as single-walled and multiwall nanotubes. Their potential as a superior adsorbent for heavy metals has been studied widely (Ahmaruzzaman, 2011; Lu & Chiu, 2006). Currently, scientists are focusing on nanomaterial synthesis which shows specific adsorption towards a single solute in the target material and ignoring the interaction potentials between mixtures that may affect the adsorption process. Carbon nanotubes have been used as a better adsorbent for the removal of heavy metals like Pb2+, Cd2+, Ni2+, and Cu2+ (M, 2010). The mechanism involved in heavy metal removal by carbon nanotubes comprises electrostatic attraction, adsorption, precipitation and chemical interaction between the metal ion and functional group present on the surface of carbon nanotubes. The drops in pH of the solution, when metal binds to carbon nanotubes indicate the transfer or release of the proton from carbon nanotubes where metal binds to it. Sometimes phenolic groups are also involved in heavy metal binding to carbon nanotubes. Moreover, an increase in metal ion concentration also increases the pH values of the solution and indicate the adsorption of heavy metal (Park et al., 2005; Zhang, 2003). Various carbon nanotubes have been synthesized to improve adsorption capability and minimize weaknesses under different environmental circumstances, for the removal of heavy metals. The combination of magnetic properties of iron oxide with adsorption properties of carbon nanotubes may prove rapid, effective and promising technology for hazardous heavy metals removal. The maximum adsorption capacity of different heavy metals on carbon nanotubes differs among different heavy metals.
Table 5
Plants utilized for nanoparticle generation and their role in bioremediation of heavy metals.
Plant Species used for Nanoparticle generation | Best Heavy metal Bioremediation | References |
Noaea mucronata | Pb (98%), Zn (79.03%), Cu (73.38%), Cd (72.04%) and Ni (33.61%) | (Murugadoss, Rajamannan, & Madhusudhanan, 2009; Tang et al., 2012) |
Euphorbia macroclada | Pb (92%), Zn (76.05%), Cu (74.66), Cd (69.08%) and Ni (31.50%) | (M, 2010) |
Graphenes
Graphene-based adsorbents have a great adsorption capacity for removing heavy metal pollutants from water and soil (Park et al., 2005; Zhang, 2003). Many researchers are focusing on and putting their efforts into investigating the potential application of graphene oxide nanomaterials in eliminating heavy metals from water and soil. The adsorption properties of graphene nanomaterials may be improved if graphene nanomaterial is synthesized by employing the Hummers method which incorporates many oxygen-containing groups like carboxyl and hydroxyl groups on the surface of nanomaterials (Zhong et al., 2009). These functional groups increase the surface area of graphene oxide nanomaterial which further increases the adsorption capacity of nanomaterials for heavy metals. The mechanism of heavy metal removal by graphene nanomaterials is the same as was discussed for carbon nanotubes. The high dispersion nature of graphene oxide nanomaterials makes it more advantageous over carbon nanotubes. The other nanomaterials that have been studied include carbon fibre, carbon, nanoporous carbon and their complexes (Prabhu & Poulose, 2012). They show exceptional adsorbent capacity for heavy metal removal and the rates of removal are persistent with pollution control requirements. Their efficiency depends on well-developed internal pore structures, large specific surface areas, and the presence of functional groups on surface nanomaterials.
Other nanomaterials
Along with carbon-based nanomaterials, other nanomaterials such as iron-based nanomaterials, photocatalytic nanomaterials, silica, alumina, and manganese dioxide nanomaterials are also explored for heavy metal pollutant removal. These nanoparticles have been engrossed for their outstanding adsorption properties. Some other nanomaterials which are utilized in the removal of heavy metal from soil, air and water are TiO2 -Based Nanomaterials, ZnO –based nanomaterials, Iron oxide nanomaterials, bimetallic nanoparticles, and Metal based-nanomaterials (Pejman, Bidhendi, Ardestani, Saeedi, & Baghvand, 2015). All possess excellent efficiency for the removal of heavy metal pollution from agricultural lands and irrigation water. But still, a lot of further research is required on nanoscience to remove heavy metals more efficiently and significantly from agricultural lands and water.
Nanotechnology has an enormous role in the field of nano-fertilizer. Nano-based fertilizer can do balanced nutrient supply, stimulate crop growth, improve crop quality, regulate nutrient migration to the environment, reduce of cost of production due to its less requirement per hectare, helps in precision farming, increase nutrient use efficiency of plants, increased drought and salt stress tolerance to plants (Kalia, Sharma, Kaur, Harleen, & Kaur, 2020; Mikula et al., 2020). The two most important applications of nanotechnology in nano-fertilizers include the controlled release of fertilizers and the bio-availability of nutrients (Rehmanullah et al., 2020). Apart from these two roles nanotechnology can also be used for the target modulation and regulation of Plant Growth-Promoting Rhizobacteria (PGPR) at the genetic or proteomic level (Fu, Wang, Dhankher, & Xing, 2020). In India, nano-fertilizers are used mostly to boost zinc uptake in wheat and other cereals, but there is a gap in research and its application by the farmers. Agriculture is facing various challenges such as climate changes, environmental issues, urbanization, sustainable use of resources and accumulation of pesticide and fertilizers. More research and work is needed to make these solutions possible at ground level i.e. to the farmer’s level. There should be subsidized programs on the use of these technologies by the farmers so that maximum benefits of these technologies can be seen on ground-level reality. All farmers should be made aware of the use and benefits of these products for their crops and particular farming process. Without these efforts, the gap between research and its application by the farmers cannot be filled. The application and challenges of nanotechnology for sustainable agriculture in India can be found in the referenced review (Salama, El-Aziz, Rizk, Elwahed, & A, 2021). The impact of different nano-fertilizers on the yield and growth characteristics of particular crops is illustrated in Table 7; Table 6 below.
Nanotechnology has a promising approach in controlling the diseases of crops. It is beneficial over conventional approaches, such as reduced input requirements, better efficacy and lower eco-toxicity. Nanomaterials may act as antimicrobial agents, biostimulants that induce plant innate immunity, and as carriers for active ingredients such as elicitors, micronutrients, and pesticides in controlling diseases (Ranjan et al., 2021; Usman et al., 2020). Thus nanotechnology may provide potential benefits in the field of disease management and agricultural adaptations shortly.
The use of nanomaterials as agrochemicals either as fertilizers or pesticides is scientifically being explored before it could be used in agriculture for a general farm practice. The properties of nanomaterials such as type of material, shape, size, crystal phase, solubility, exposure and dosage concentrations are considered to be of the potential risk to human health (Pitambara, Shukla, Panpatte, & Jhala, 2019). However, some experts point out that food products containing nanomaterials available in the market are probably safe to eat, then also this area needs to be investigated more actively because detailed studies are required to address the impact and safety concern of nanomaterials within the human body when exposed to nanomaterials via food (Guha, Gopal, Kundu, & Mukherjee, 2020).
Table 6
Impact of Nano-fertilizers on yields of different crops under varying climatic conditions (Hasanuzzaman, Fujita, Filho, Nogueira, & Galindo, 2020).
Table 7
Impact of different nano-fertilizers on different growth and survival characteristics of particular crops (N et al., 2018).
Researchers have to develop proper assessment approaches to assess the impact of nanomaterials or nano-fertilizers on biotic and abiotic components of the ecosystem (Heinisch, Jácome, & Miricescu, 2019). Amongst the different issues concerning the use of nanomaterials in agriculture, the nature of accumulation of nanomaterials in the environment and the focus on the edible part of plants might be the important issues before their use.
Nevertheless, the impending use of nanotechnology in the fields of food, agriculture, nano-fertilizer, plant nutrition and plant protection suggested that nanoparticles may be toxic to the environment and biological system due to their changed physicochemical properties (Ghorbanpour, Bhargava, Varma, & Choudhary, 2020). Thus while considering the benefits of nanomaterials researchers should also focus on the probable risk that may arise due to that nanomaterials.
Role of nanoparticles in Precision farming
Advanced biosensors for precision farming are possible only by nanoparticles or nanochips. Modern nanotechnology has the potential to address the problems of conventional agriculture and can revolutionize this sector. The potential uses and benefits are enormous that including insect pest management via formulations of nanomaterial-based pesticides and insecticides, increase in agricultural productivity using nanoparticles encapsulated fertilizers for slow and sustained release of nutrients and water. Precision agriculture uses AgTech to collect detailed field and crop data, mainly using sensing technologies combined with geo-referencing. Over the last few decades, many new technologies have been developed for precision farming (Duhan et al., 2017). Some of these are satellite positioning (GPS) systems, remote sensing, automated steering system, geo-mapping and variable rate technology (VRT). Some other roles of nanoparticles in precision farming are listed in Figure 5.
Applications of Nano-based sensors in agriculture
Presently, the role of Nano-based sensors in Agriculture is more captivating over traditional biosensors, in enhanced detection sensitivity/specificity. Some of the potential applications of Nano-based sensors are listed below:
Nano-based sensors are used in diagnostic tools utilized in quality and disease assessment of soil
Soil diseases caused by infecting soil micro-organisms like viruses, bacteria, and fungi may be assessed by Nano-based sensors via the quantitative measurement of differential oxygen consumption of “good microbes” and “bad microbes” in the soil (relative activity). The oxygen consumption data of two microbes can be determined by employing two sensors impregnated one with “good microbes” and the other with “bad microbes” respectively, are immersed in a suspension of soil sample prepared in a buffer solution. In this way by comparing the data from two different samples, we can easily decide which microbe favours the soil. We can also predict whether or not soil disease is ready to break out in the tested soil beforehand. Thus the nano-based sensor proposes an inventive technique of diagnosing soil conditions (Parashar et al., 2009).
Nano-based sensors act as an agent to promote sustainable agriculture
In this regards Nano-fertilizers are used to maintain nitrogen levels of soils by long-term assimilation by soil microorganisms and preventing nitrogen loss due to leaching, emissions (Boruah et al., 2012). Nano-fertilizers are products that carry nutrients encapsulated within a nanoparticle. Encapsulation of nutrients is carried out in three ways: firstly, the nutrient is encapsulated inside nanomaterials called nanotubes or Nano-porous materials; secondly, the nutrient is coated with a thin protective polymer film; thirdly, the nutrient is divided into particles or emulsions of nanoscale dimensions, so that they can easily penetrate the tissues. Nano-based carbon tubes have been shown to penetrate tomato seeds and zinc oxide-based nanoparticles have been shown to enter the root tissue of ryegrass (Dwivedi et al., 2011; Fazaludeen et al., 2012). In this way, new nutrient delivery systems on plant surfaces can be developed. However, to achieve sustained release of nutrients on-demand and prevention of conversion of nutrients to non-absorbable forms by plants, a biosensor could be attached to this Nano-fertilizer that allows selective nitrogen release linked to time, environmental and soil nutrient condition signals. It decreases toxic effects of soil associated with fertilizer over application.
Zeolites (naturally occurring crystalline aluminium silicates) maintain plant growth and yield by improving long term soil quality and reducing the loss of nutrients in the soil. It shows properties to hold nutrients in the root zone of plants to use when required. This helps in the efficient use of nitrogen and potash fertilizers for the same yield lasting longer. Zeolites do not break down over time and remain in the soil and improve the nutrients and water holding capacity of the soil, permanently. Zeolites with subsequent applications could be linked to a Nano-based sensor which can modernize agriculture in the sense that the deficiency of water/nutrients in either plant or soil can be sensed and regulated by the controlled release of retained water/nutrients in the zeolite. Similarly, Nano-based pesticides and herbicides are being developed that will be combined with a smart delivery system that will show a timely release system or an environmental trigger release of content (Aromal et al., 2012). It will result in increased agricultural production and less injury to agricultural workers.
Role of Nano-based sensors in devices used for contaminants detection and other molecules
Several devices have been designed based on Nano-sensors to detect contamination of pests, nutrient content and plant stresses due to temperature, pressure or drought. They are also helpful for farmers to enhance competence by applying input only when necessary. One such example is organophosphorus pesticides like dichlorvos and paraoxon which are used at very low levels that’s too in a controlled manner mediated by liposome-based biosensors (Mubarakali et al., 2011). The flow injection analysis (FIA) based method has been developed for Escherichia coli (E. coli) detection by using modified GCE bismuth Nano-film (Raghunandan et al., 2010). A biochip sensor system, consisting of two Ti contact pads and a 150 nm wide Ti nanowell device on LiNbO3 substrate has been constructed which works on voltage fluctuations system observed in the nano-well displaying a power spectral density (PSD) When the bacteria were resistant to the phages (uninfected bacteria) (Yadav et al., 2017). Photosystem II (PS II) based biosensor has been designed which is known to bind with different herbicides isolated from photosynthetic organisms may also be used to monitor different polluting chemicals in sewage sludge, groundwater, irrigation water, industrial and urban effluents. It could lead to the set-up of a low cost, easy-to-use apparatus, able to reveal specific herbicides and a wide range of organic compounds.
Nano-sensors based tools for effective detection of proteins and nucleotides
There are several Nano-sensors like ssDNA-CNTs probes, optical biosensors to detect specific kinds of DNA oligonucleotides (Gurunathan et al., 2009), Nanobiosensor with GNPs functionalized with alkane thiol-capped LNA/DNA chimaeras in a tail-to-tail hybridization mode for single-stranded DNA, MWNTs/ZnO/CHIT composite film modified GCE for immobilization ssDNA probes to effectively discriminate different DNA sequences (Gericke et al., 2006), Nanobiosensor with a bio-nanocomposite layer of MWNT in chitosan deposited on an SPCE for the detection of deep DNA damage, Nano-SiO2/p-amino thiophenol (PATP) film for the detection of the PAT gene sequences by a label-free EIS method. A nanowire field-effect transistor-based biosensor have been reported which detected simple and ultra-sensitive electronic DNA methylation and evades complicated bisulfite treatment and PCR amplification (Surette, Nason, Harper, & Mitrano, 2021). In the same way, properties of protein-ligand or antigen interaction can realize the ultra-sensitive detection of special protein molecules in protein-nanoparticles based biosensors systems. These DNA and protein detection biosensors systems might play a crucial role in the discrimination of one plant species from another, in the detection of plant pathogens. Recently prohibited antibiotics were detected reliably, effectively and in a short time in honey using biosensors based system.
The future scenario of nanotechnology in the environment and bio-sustainability
Nanotechnology is a capable domain of recent times. Metallic nanoparticles are mainly produced during enzymatic reactions. Mechanistic understandings may provide control over morphology, stability, and rate of synthesis of nanomaterials. Environmental contaminants are remediated by biologically synthesized nanomaterials (Masood & Malik, 2013). Heavy metal pollution has been seen as a significant concern because of its hazardous impacts and non-biodegradable nature (Wu et al., 2016). Bioremediation of heavy metal pollution has been preferred over other methods such as chemical precipitation and electrochemical methods (Diaz et al., 2015). Microbes have been evolved and can offer inventive bio-based clean-up methods (Engel, 2016). Metal reducing microbes promotes precipitations, minerals dissolutions and transformations such as geochemical agents (Neumann, Gulati, & Nolan, 2017). Microbial reduction methods have delivered a new way to explore nanotechnology. Bio-mineralization is among, one of them which controls the environmental impact of heavy metals (Kang, Qu, Alvarez, & Zhu, 2017). The enzyme metal interaction here provides detoxification of heavy metals by a biotransformation process. Microbes that produce nitrate reductase can enhance the efficacy of the bioremediation process. Other enzymes along with nitrate reductase have been studied for further possibilities of heavy metal remediation. Microbe metal interaction and the importance of their enzymes still required further investigation (Waseem et al., 2019). The studies of these microbial interactions at the genetic level may provide new genetic tools for bioremediation. These findings may assist in clarifying new phenomena such as antibiotic resistance (Ghosh et al., 2012). The upsurge in antibiotic resistance is becoming a serious concern all over the world for modern medicine. The combination of metallic nanomaterials with antibiotics may overcome these problems such as bacterial resistance (Jang et al., 2018). However, the co-occurrence of antibiotic resistance and metallic resistance genes have been reported recently. Thus microbes may play a critical role in controlling heavy metal pollution. The use of microbial nanomaterials for renewable energy needs further investigation.
Safety recommendations of Nanoparticles use in agriculture and environment
Nanoparticles have promising applications but they should be developed safely. Their safe, use and disposal at the end of their life must be taken into account. However, it cannot be overlooked that most of the knowledge we gathered about it is mainly from laboratory experiments. Thus it is almost uncertain about apprehending the practical application of it while understanding its impact on environment-related toxicity. For the most part, no one knows and not having the guidelines, regulatory bodies, and similar organizations create obstructions. Hence, due to the perceived gaps of knowledge, there is a need to execute large trials in forwarding and developing futuristic investigations. However, not all manufactured nanomaterials are used similarly for all applications, some might bear a higher hazard potential than others, and this scenario could lead to different exposure probabilities. To improve both the safety and efficacy of nanotechnology, a new proactive approach is necessary, based on pre-regulatory safety assessment and dialogue between stakeholders (Bundschuh et al., 2018). In this context following safety recommendations should be followed:
1. Comprehensive knowledge should be developed about what property makes a nanomaterial or Nano-product more or less safe and how to implement this knowledge in a structured way into industrial innovation processes and information exchange between the involved stakeholders.
2. It should be counted that the distinguished properties of Nanomaterials, such as magnetic, optical, or chemical properties, makes them useful for a broad range of applications in various fields. These properties determine the physicochemical properties of nanomaterials like composition, size, crystallinity and surface. They are the basis for applications of manufactured nanomaterials.
3. Use of green chemistry is a way to maximize the functionality of manufactured nanomaterials by reducing the presence of inactive components, and at the same time to develop a synthesis process as simple and sustainable as possible.
4. To maximize functionality, nanomaterials or Nano-products should not affect human health nor impact the environment along their life cycle. As safety is regarded as the reciprocal of risk, which is a measure of the probability that harm will occur under defined conditions of exposure to a nanomaterial or Nano-product.
5. Aspects of nanomaterial's safety have to consider both hazard, which is the potential of a nanomaterial to cause damage, and exposure, which includes that a certain dose encounters human beings or the environment over time.
6. The strategies for safe based design first is to reduce hazard and second is to reduce exposure mediated by the release of nanomaterials during the life cycle.
7. The implementation of the safe based design is based on knowledge of the relationship between designed manufactured nanomaterials properties, their interactions and effects at the cellular and body level. So, the initiated toxicological response, as well as potential release and exposure scenarios, should also be considered.
8. To avoid some of the problems, it is important to take public views on Nano-based materials into account at an early stage of product development.
9. Depending on the properties of a particular product, the risks associated with dose and exposure should be assessed properly that allows one to predict what happens when these very small materials, designed to be biologically active, enter the human body or are dispersed in the environment.
10. It is also important to recognize, that as new particles and applications are developed, and as more information becomes available on fate, behaviour, routes of uptake and entry into the atmosphere, these predictions may change. Moreover, the nanomaterials once entered into the environment have the potential to accumulate in the environmental organisms.
The fate of Nanoparticle in the natural geochemical cycle and their removal from the environment
Nanomaterials produced artificially can also be formed naturally in soil, aerosols, waters, deep-sea hydrothermal vents, natural ore and microbial systems. Even with many reports on the natural occurrence of inorganic nanoparticles, the unveiling of their formation mechanisms is still unclear because of lacking detailed mechanistic studies on mechanical, thermal, chemical, photochemical and biological processes delivering natural nanoparticles. Moreover, more than one reaction pathway may contribute to the formation of natural nanoparticles in the environment. To measure the fate of nanoparticles, the role of phase boundaries, reaction conditions and the microcrystalline substrate should be elucidated. Very little is known about how Nanoparticles interact with the environment. Nanoparticles exhibit varying toxicity, depending on the functionality of the organic matter and the generation of reactive oxygen species. Therefore future studies should be envisaged to evaluate the underlying mechanisms of toxicity of Nanoparticles. Considerable progress regarding the understanding of sources, fate, and effects of nanoparticles has been made over the last decade. Considerable progress regarding the understanding of sources, fate, and effects of nanoparticles has been made over the last decade and simultaneously, increasing attention on the effects of nanoparticles on aquatic and terrestrial systems have been received. Environmental concentrations based on modelling approaches could be confirmed by measured concentrations in the field. Nonetheless, analytical techniques are, still under development to more efficiently and reliably characterize and quantify nanoparticles, as well as to detect them in complex environmental matrixes. Spell the debate on the relevance of nanoparticle-released metal ions for their toxicity is still ongoing, it is a re-occurring phenomenon that how inert-nanoparticles can interact with biota through physical pathways such as biological surface coating. This interferes with the growth and behaviour of organisms and there are multiple shreds of evidence suggesting nanoparticles as a sink for organic and inorganic co-contaminants (Sharma et al., 2015). Nanoparticles can enter the environment along their life cycle and generally three emission scenarios are considered: (i) release during the production of raw material and Nano-enabled products; (ii) release during use; and (iii) release after use of Nanomaterial containing products. So far, emission and environmental concentration levels of the Nanoparticle life cycle have been estimated using material flow models. Calculation models assume that produced Nanoparticles are released either to waste streams or directly to environmental compartments, and more realistic approaches account for the delayed release during the use of Nanoparticle stocks. Nanoparticle emissions are also controlled by (i) ageing or weathering (ii) the fate of the Nanoparticle during use, and (iii) the waste management system. However, production volumes may give a good indication of the emission of Nanoparticle. Therefore, to understand Nanoparticle's fate, thorough characterization of Nanoparticle surface chemistry i.e. the formation or loss of coating over time seems unavoidable but it is still hugely exacting (Surette et al., 2021). Alterations in chemical speciation, dissolution, degradation, as well as alteration of the surface properties by precipitation and ad- or desorption are important chemical transformation processes of Nanoparticle, which are frequently been investigated both in aquatic and soil ecosystems and can be castoff for confiscation. However, specifically focusing on dissolution, passivation, aggregation, adsorption, sedimentation, and deposition are the most relevant processes for confiscation under field conditions. The colloidal stability, chemical transformation and transport of Nanoparticles through porous media are some of the key factors controlling their fate, effects and confiscation. When Nanoparticles are released into the environment, they interact with a variety of dissolved or particulate, inorganic or organic compounds sway their aggregation dynamics and colloidal stability. By focusing on factors controlling homo-aggregation and hetero-aggregation as well as disaggregation, we can determine Nanoparticles fate (Yaqoob et al., 2021). The dissolution of Nanoparticles is driven directly by particle chemistry, which suggests that the scientific community is indeed capable of advancing the knowledge that allows for reasonably accurate predictions and modelling. In porous media, ionic strength prompts the Nanoparticle's fate. Nanoparticles retention and deposition in porous media are related to the ionic strength of the pore water, which drives quick Nanoparticles aggregation. In summary, predominantly qualitative and quantitative information about Nanoparticle is necessary to know their availability and fate in the geochemical cycle or environment (Samuel et al., 2021). Environmental key factors controlling Nanoparticles fate processes are ionic strength, type and charge of ions, pH, type and concentration of natural organic matter in all environmental compartments, while the degree of water saturation is an additional factor for porous media. These aspects should be addressed in future work. There is no generic mechanism considered for all Nanoparticles' fate and confiscation. In this regard the Key learning points are as follows:
Irrespective of human activities, naturally occurring nanoparticles are often present in all spheres of the Earth (i.e., in the atmosphere, hydrosphere, lithosphere and even in the biosphere).
Natural organic matter largely contributes to the fate of metal nanoparticles, in the environment
Mechanistically, the formation of metal nanoparticles results in the reaction of reactive oxygen species and Natural organic matter complexes with dissolved metal ions and this reaction is enhanced by elevated temperature and/or exposure to light.
Water properties such as pH, redox conditions, ionic strength, and gathering of various natural organic matter, determine the growth and stability of nanoparticles in the aquatic environment.
Organic matter-coated nanoparticles display less toxicity than inorganic nanoparticles that are surface-coated by polymers and/or surfactants.
Conclusion
Development in engineering and nanoscience technology may prove more helpful for generating new chances to develop more cost-effective and environmentally safe agricultural technologies. The nanoparticle has peculiar properties which make them more suitable to solve pollution problems. Current findings have indicated that nanomaterials are beneficial particles for heavy metal removal because of their unique and efficient properties in removing heavy metals from the agricultural system. The microbial threats to agricultural quality and production may also be removed with the help of nanotechnology. Zinc, chitosan and copper-based nanomaterials possess antimicrobial and antifungal properties which in turn can solve these microbial problems in agriculture and may increase agricultural production. Thus further research must be made in this direction to develop nanoparticles with greater stability, specificity and capability for simultaneous removal of multiple contaminants, and microbial pathogens from agriculture. It is not clear that why and how different species of plants show dissimilar resistance properties to nanoparticles. It is recommended to discover the effects of environmental factors on the uptake and accumulation of nanoparticles by different plant species. The route of nanoparticles movement in plant’s cells and their effect on the genetic system of plants should be investigated.