Introduction
In mammals, the body's hemostatic system provides host protection by creating a closed circulating system of high-pressure and smooth blood flow without any interaction of blood cells with the blood vessels. The blood vessel is tiled with endothelial cells and is responsible for maintaining an inert and passive surface for the whole circulating system. Together with blood vessels and their cellular architecture, various cellular components of blood, along with leukocytes and platelets, derive microparticles that circulate through this closed system in an inert and inactive state and participate directly in maintaining the hemostasis. In response to cellular injury or tissue disruption, the hemostatic system and endothelial cells are activated, which initiates the expression of intracellular intercellular adhesion molecule-1 (ICAM-1), ICAM-2 and ICAM-3, vascular and platelet adhesion molecules, and Weibel–Palade bodies on the endothelial cell membrane. In response to pathological events in arteries and veins, the exposure of the endothelial surface to negatively charged blood phospholipids can interfere with tissue and coagulation factors, therefore promoting the prothrombotic signaling pathways to repair the changes within the plasma membrane architecture. The release of tissue and coagulation factors from the plasma membrane bilayer of injured endothelial cells activated a calcium-dependent enzymatic reaction to convert the inactive blood clotting protein (zymogens) to corresponding active enzymes, resulting in rapid generation of thrombin which further initiates thrombus formation (Furie & Furie, 2007; Lippi, Franchini, & Targher, 2011).
Thrombus formation on the disrupted atherosclerotic plaque is one of the critical factors for developing ischemic cardiovascular disorders (Asada, Yamashita, Sato, & Hatakeyama, 2018). On the other hand, loss of integrity in the endothelium of brain-supplying arteries triggers platelet aggregation pathways, stimulation of the coagulation cascade, and changes in the erythrocyte or leukocyte constituents of the blood, resulting in thrombotic occlusion and cerebral ischemic tissue injury (Hacke, Zoppo, Del, & Harker, 1987). Antithrombotic drugs in routine use include antiplatelet drugs (aspirin, clopidogrel, and glycoprotein IIb/IIIa receptor antagonists) and anticoagulants (unfractionated and low molecular weight heparin, warfarin, and direct thrombin inhibitors). Antithrombotic drugs, which include antiplatelet and anticoagulant therapies, prevent, and treat many cardiovascular disorders and, as such, are some of the most commonly prescribed drugs worldwide. The first drugs designed to inhibit platelets or coagulation factors, such as the antiplatelet clopidogrel and the anticoagulant warfarin, significantly reduced the risk of thrombotic events at the cost of increased bleeding in patients. However, both clopidogrel and warfarin have some pharmacological limitations, including interpatient variability in antithrombotic effects in part due to the metabolism, interactions (e.g., drug, environment, and genetic), or targets of the drugs (Mega & Simon, 2015; Watson, Chin, & Lip, 2002). Therefore, it becomes essential to search for new antithrombotic agents having minimum adverse reactions. Medicinal plants and plant-derived natural bio-active compounds may serve crucial roles in this regard (Chen et al., 2015).
People are primarily inclined to natural traditional healing agents to maintain their health and as a source to diagnose, prevent, improve, or treat physical and mental ailments based on their beliefs, theories, and perceptions indigenous to different cultures (Che, George, Ijinu, Pushpangadan, & Andrae-Marobela, 2017). Multiple lines of research are going on to find natural remedies against various chronic diseases based on the traditional use of indigenous people. Isolation of bioactive compounds and bioactive compound-based analysis are effective ways to prove the conventional efficacy of medicinal plants. Dendropanax morbifera Leveille is a Korean endemic tree species that people of the Republic of Korea have consumed for a long time as healthy food and traditional medicine; it is historically used to improve blood circulation, which is mentioned in Donguibogam (a precious book of Korean medicine written in the 17th century) and for the treatment of various other diseases (Choi et al., 2015). Celastrus orbiculatus Thunb (C. orbiculatus) is a traditional Chinese phytomedicine applied to relieve pain, improve blood supply, tranquilize, and mitigate excitement for centuries. This plant is auspicious with various bioactive compounds and proves its traditional effectiveness in different experimental set-ups (Zhou et al., 2019). A combination of four-herbal formulas from Radix astragali, Radix scrophulariaceae, Panax notoginseng, and Salvia miltiorrhizae develop a capsule formulation named Fufang Xueshuantong (FXST), which is well appreciated in Chinese medicine because of its effectiveness in the management of clinical cardiovascular disorders (Sheng et al., 2014). Another commonly used Chinese traditional medicinal herb named Danggui (Radix Angelicae Sinensis) and Honghua (Flos Carthami Tinctorii) are well recognized for stimulating blood supply and eliminating blood stasis. It has been reported that these two medicinal herbs potentiate the activity of clopidogrel (an antiplatelet medication) by prolonging bleeding times (Li & Wang, 2010). Plant-derived natural bioactive compounds, including polyphenols, flavonoids, alkaloids, glycosides, polysaccharides, etc., possess the potential to maintain mitochondrial protein expression, oxidative phosphorylation, and regulate the production of ROS (reactive oxygen species). These properties make them potential candidates as cardioprotective agents (Arauna et al., 2019). Hovertrichoside C, hyperin, luteolin-7-O- -D-glucuronide, pentacyclic flavonol, quercitrin and avicularin, hexacyclic flavonol (Lee, Son, & Han, 2002), sesquiterpenes (Raskob et al., 2014) isolated from various plants and plant parts have been reported to produce antithrombotic and antiplatelet aggression activities through the inhibition of TFs activities. Hyaluronan (HA) polysaccharide, plasma free hemoglobin (PFH), hyperoside, withaferin A, wogonin (WGN), etc., induce anticoagulant activity by suppressing the intrinsic or extrinsic coagulation pathways (Chen et al., 2015).
This review will introduce the readers to the current therapies and their drawbacks and how the novel plants themselves or their bioactive compounds can manage the thrombosis complication in different cardiovascular disorders more efficiently with their probable mechanisms in light.
Epidemiological status of cardiovascular disorder
Worldwide, cardiovascular, and cerebrovascular diseases are the leading causes of mortality, morbidity, and disability. It has been reported that the formation of thrombus into the vessels is considered the principal cause of the development of the acute brain and coronary artery occlusion and plays a cardinal role in the pathology of stroke, ischemic heart disease (IHD), and venous thromboembolism occurrence (Pifarre, 1998; Raskob et al., 2014). In the developed world, arterial and venous thromboembolic events, including deep vein thrombosis (DVT), acute coronary syndrome, strokes, pulmonary embolism (PE), and peripheral arterial thrombosis, is the most prominent causes of death than any other pathological complications. Various angiographic and pathologic data demonstrated that acute MI (myocardial infarction) and unstable angina are present with intraluminal thrombi, where the prevalence of thrombi in acute myocardial infarction is high (Pifarre, 1998). So, minimization of thrombus formation is one of the main prerequisites for the treatment of MI. Every year, an approximate count of 17 million people die from various cardiovascular and cerebrovascular complications. Among them, myocardial infractions and strokes contribute enormously to global morbidity and mortality and are recognized as significant barriers to sustainable human development (Richling et al., 2007). Globally, one in four deaths has been reported as a collective consequence of IHD and strokes (Lozano et al., 2012). Thrombus formation contributes to nearly half of all ischemic stroke deaths, and this death rate is alarmingly increasing. Globally, individuals over 75 years old experience 60% of new stroke, and 45% of death occurs from strokes in this age range (Feigin et al., 2014). A study of GBD (Global Burden of Diseases, Injuries, and Risk Factors) presented unambiguous evidence regarding the negative impact of arterial thrombosis on global health since arterial thrombosis covertly controls the pathologic mechanisms responsible for IHD and ischemic stroke development (Raskob et al., 2014). So, to avoid severe complications from intraluminal thrombus and embolus, it is medically recommended to treat the patients with anticoagulant therapies as quickly as possible with an adequately adjusted dose and dose frequency. The primary function of currently available antithrombotic agents is to prevent the formation of blood clots. Most of the current antithrombotic agents are functioning by inhibiting platelet function directly (for example, aspirin, clopidogrel, and dipyridamole) or, via thrombin inhibition, by inhibiting platelet activation and fibrin formation (for example, heparins, warfarin and direct inhibitors of thrombin or factor Xa) (Mega & Simon, 2015).
Cardiovascular disorder and thrombus formation
Blood coagulation is crucial in reducing blood loss from vascular wall injury in the body. Hemostasis is of Greek origin that is characterized as the stop of hemorrhage. The hemostasis mechanism reflects a complex and responsive balance between blood clotting and the breakdown of fibrin polymers (Thornton & Douglas, 2010). Thrombus formation happens with the aid of blood vessels, platelets, and coagulation factors. When the damage to endothelial cells occurs, the platelets bind to the collagen located on the sub-endothelial sites and develop a short plug. This plug forming also induces coagulation cascades, which form a stable platelet plug by activating fibrin (Norris, 2003). The process of hemostasis occurs in four stages: vascular spasm, platelet aggregation, platelet plug formation, coagulation cascades activation, and stimulation of the breakdown of fibrin threads (Gale, 2011).
A vascular injury immediately triggers vasoconstriction governed by responsive neurologic pathways and discharges of specific chemicals such as endothelin and thromboxane A2 (TXA2) to reduce blood flow through the affected area (Cines et al., 1998). Collagens and von Willebrand factors (vWF) present in the sub-endothelial sites are revealed due to the breakdown of endothelial cells. Then platelets bind with collagens through glycoprotein-1b (GP1b) complex receptors with the help of vWF, causing structural changes in platelets, leading to multiple pseudopods, and significantly maximizing their outer region. This change indicates the activation of platelets (Berndt, Shen, Dopheide, Gardiner, & Andrews, 2001). Activated platelets secret various chemicals from two types of granules. α granules are present with fibronectin, fibrinogen, platelet factor IV, factor V, factor VIII, and platelet-derived growth factor. In contrast, dense granules are present with a massive number of Ca2+ and adenosine diphosphate (ADP). ADP and TXA2 promote the addition of more platelet, which induces the development of a platelet plug that transiently restricts vascular damage (Figure 1) (Heemskerk, Bevers, & Lindhout, 2002).
Figure 1
Mechanism of thrombus formation. RBC= red blood cell; TXA2= thromboxane A2; 5HT= 5-hydroxytryptamine receptors; VWF= von Willebrand factor.
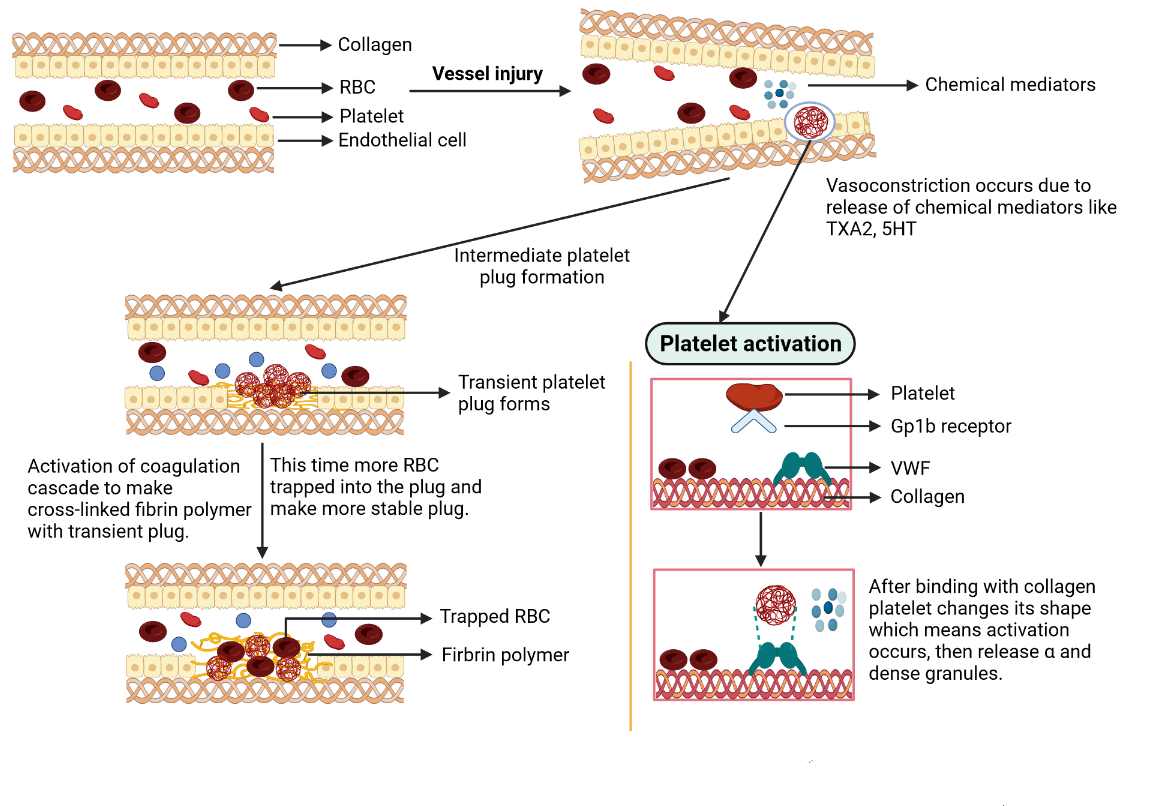
Vascular damage triggers coagulation by activating coagulation cascades to create a more stable platelet clot. This process occurs in three ways, e.g., extrinsic, intrinsic, and common pathways (Figure 2). The extrinsic or tissue factor pathway is first activated by the tissue factor (TF), which is liberated from the sites of the sub-endothelium (Bugge et al., 1996). In the case of sub-endothelial cell exposure, TF reveals in plasma, binds with factor VII, and converts into TF-VIIa complex, which also helps transform both factors IX into IXa and X into Xa (Morrissey, 2001). Initiation of intrinsic pathways activates factor XII that is converted into XIIa with the help of high molecular weight kininogen (HMWK), and plasma kallikrein, prekallikrein (PK) act as a precursor of plasma kallikrein (Colman & Schmaier, 1997). Factor XIIa also instigate the transformation of factor XI to XIa and prekallikrein to kallikrein. In this activation reaction of factor XIa, Ca2+ ions are required (Bouma & Griffin, 1977; Rasche, 2001). Activated factor XIa stimulates the conversion of factor IX to the activated form of IXa, mediated by Ca2+ ions and the TF-VIIa complex (Bouma & Griffin, 1977; Grover & Mackman, 2019). It also helps activate the factor X by converting it into Xa with the help of phospholipids (PL), Ca2+ ions, and the activated form of co-factor VIIIa (Borne, Meijers, & Bouma, 1995). An activated form of VIIIa and IXa is known as “the tenase complex.” (Norris, 2003) This activation form of factor Xa can be acquired from the extrinsic pathway. The activation process of factor X is also known as the initial step of the common pathway. In this pathway, Xa activates thrombin from prothrombin with the help of PL, Ca2+ ions, and the activated form of co-factor Va. Thrombin which is also recognized as an influential enzyme that catalyzes the conversion of fibrinogen into fibrin monomer by the cleavage process. This fibrin then links with one another to form a fibrin polymer. Thrombin also helps to convert factor XIII into the activated form of XIIIa (Brummel, Paradis, Butenas, & Mann, 2002), which further facilitates the formation of a stable cross-linked fibrin polymer by providing additional bonding between fibrin polymers. Thrombin produces positive feedback in enhancing the conversion of factor XI to factor XIa (Gailani & Broze, 1991) and stimulates the activation of co-factor V to Va and VIII to VIIIa (Brummel et al., 2002). Thrombosis is considered one of the major risk factors for developing three leading CVDs: venous thromboembolism, (Gregson et al., 2019) IHD, and stroke (Raskob et al., 2014). Atherothrombosis develops when the atherosclerotic plug is disrupted, a recurrent condition that decreases blood flow passage into the artery (Figure 3). As a result, MI or ischemic stroke are seen for this atherothrombosis reason (Cate & Hemker, 2016). Patients with a high level of cholesterol and other fatty substances accumulate these into the damaged endothelial cells of the artery wall. Oxidation of cholesterol causes an inflammatory response that triggers white blood cells to enter the artery wall and transform into macrophages. This formation of white blood cells (WBC) digests sub-endothelium deposited cholesterol and turns into foam cells (Tedgui & Mallat, 2006). Within the artery wall, foam cells degenerate and release their contents, forming the atheroma. Calcium salts, smooth muscle cells, and collagen matrix accumulate within the atheroma and generate a hard plaque that reduces the elasticity of the artery wall and artery lumen (Hansson, 2005). When this plug ruptures, exposing collagens and TF that facilitate platelet aggregation and activation of coagulation cascades to generate a cross-linked fibrin platelet plug. This cross-linkage traps red blood cells, restricting the blood flow in the artery or breaking off and traveling elsewhere in the body, forming blockages where arteries narrow (Davì & Patrono, 2007). However, several studies asserted that natural products from both plant and animal sources could significantly contribute to treating cardiovascular diseases. For instance, orientin, resveratrol, tea polyphenols, curcumin, and allicin, are well-known bioactive compounds isolated from various natural extracts. These promising natural compounds defend against various cardiovascular diseases through their lipid-lowering potentials via the minimization of oxidative stress (Li, Cai, Zheng, Sun, & Ye, 2019; Lu et al., 2012; Yang et al., 2014; Yokozawa, Nakagawa, & Kitani, 2002) . It has been reported that a combinational treatment of Coelatura aegyptiaca extract and lipid-lowering drug atorvastatin increased hypolipidemic efficacy and minimized the undesirable adverse effects of the drug (Mohamed, Ibrahim, Zaki, Ali, & Soliman, 2019) . On the other hand, various bioactive compounds of animal origin also play a significant role in reducing cholesterol levels. For instance, in common dietary products, L-carnitine, including fish, meat, and milk, reduces oxidized low-density lipoprotein (LDL) cholesterol levels (Malaguarnera et al., 2009). Choline is another important nutrient widely distributed in various food items, including fruits, vegetables, fish, meat, eggs, and dairy products. Studies reported that this auspicious natural compound plays a remarkable role in lipid regulation and cholesterol metabolism (Rajabi et al., 2014; Sivanesan, Taylor, Zhang, & Bakovic, 2018). Teprotide is a simple nonapeptide that was isolated from the venom of Bothrops jararaca viper and was the lead compound to develop anti-hypertensive medications named captopril and cilazapril (Herzig et al., 2020).
Figure 2
Schematic representation of intrinsic and extrinsic pathway of the coagulation cascade and the fibrinolytic system. HMWK= high molecular weight kininogen; tPA= tissue plasminogen activator; TNF= tumor necrosis factor; PAI-1= plasminogen activator inhibitor-1;uPA: Urokinase plasminogen activator.
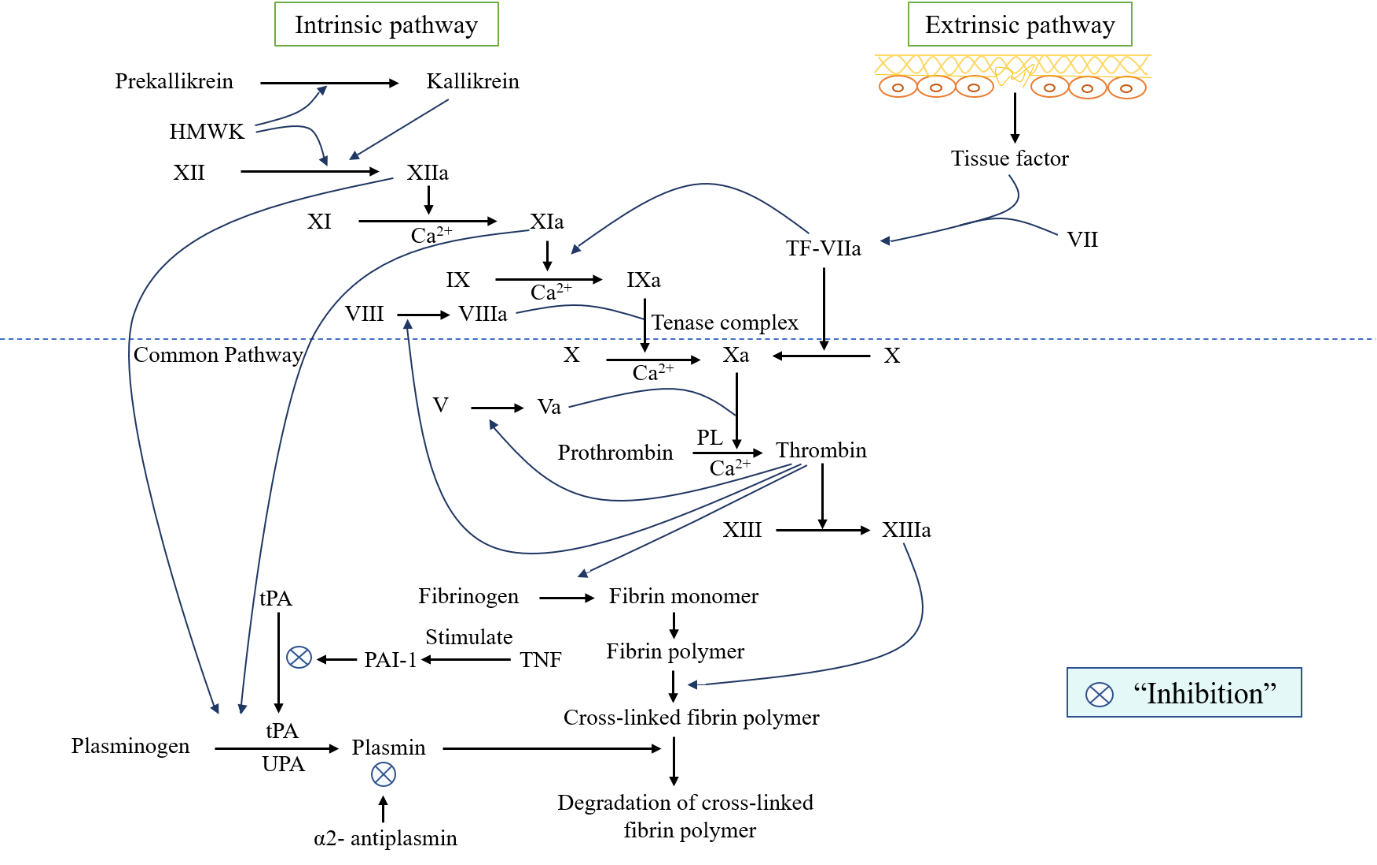
Current therapies for the treatment of thrombosis in cardiovascular disorder
Thrombosis, described as localized blood clotting in the arterial or venous circulation, has had a severe medical effect on global public health. MI or heart attack is the most major consequence of arterial thrombosis, and approximately 80% of strokes result from arterial thrombosis; these two collectively become a vital threat for the developed world population. On the other hand, venous thromboembolism occurs as the third major cause of death due to CVDs (Mackman, 2008). Various treatment strategies are available against thrombus-induced CVDs with various mechanisms of action. Antiplatelet drug therapies are administered to reduce thrombus formation in patients present with acute thrombotic events, demonstrating their action by disrupting the platelet function in normal blood clotting and interfering with platelet aggregation and activation, further increasing the risk of bleeding episodes (Meadows & Bhatt, 2007). Administration of glycoprotein IIB (GPIIB)–GPIIIA, specifically αIIbβ3-integrin, which is a platelet integrin complex, as a short-term treatment strategy for patients with acute coronary syndromes who are experiencing percutaneous coronary intervention, has been believed to suppress platelet aggregation through encumbering the binding of activated platelets to fibrinogen as well as other ligands (Kong et al., 1998). ADP receptor P2Y12 is another important target of antiplatelet drugs to reduce platelet aggregation and activation. Ticlopidine, clopidogrel, and prasugrel are the most commonly used antiplatelet drugs that antagonize the P2Y12 receptor to inhibit platelet aggregation (Gachet, 2005; Mackman, 2008). Platelet cyclooxygenase 1 (COX1) inhibitors suppress the synthesis of TXA2, which is recognized as a potent activator of platelet, and through this kind of inhibition, aspirin produces antiplatelet action (Hennekens, Sechenova, Hollar, & Serebruany, 2006). Patients with atrial fibrillation generally administer anticoagulant therapy to protect against venous thromboembolism and long-term ischemic stroke. These drugs generally target a coagulation cascade to exert anticoagulant action. Warfarin, and rivaroxaban, are used as an anticoagulant to prevent venous thromboembolism (Mackman, 2008; Weitz & Linkins, 2007). Apart from all these established therapies, several new antithrombotic drugs are in the developmental stage, and research is ongoing to evaluate their safety and efficacy. For instance, terutroban and picotamide are two new antiplatelet drugs that inhibit platelet aggregation by antagonizing the TXA2 receptor. Vorapaxar and atopaxar induce antiplatelet action by inhibiting protease-activated receptor-1 (PAR-1). Various new anticoagulants, including tifacogin, pegnivacogin, idraparinux, idrabiotaparinux, semuloparin, recomodulin, solulin, pegmusirudin, and odiparcil are also in developmental stage to facilitate the treatment strategy of thrombotic disorders more promptly (Bivard, Lin, & Parsonsb, 2013).
Currently used antithrombotic drugs and their potential side effects
Several classes of drugs are currently prescribed to manage thrombosis-induced cardiovascular diseases. But, most of them are associated with various side effects, including abnormal bleeding events, gastrointestinal problems, low blood platelet count, kidney problems, and so on (Figure 4). Antiplatelet medications hold back the formation central to arterial thrombus formation and therefore be an important therapy to manage cardiovascular diseases. Among the drugs of this class, clopidogrel is in the first market race. This drug irreversibly put a stop to binding ADP to its platelet receptors. ADP is responsible for activating the GP IIb/IIIa complex, the central fibrinogen receptor for platelet aggregation. Thereby, blockade of ADP receptors stands in the way of platelet activation, which further inhibits platelet activation. ADP receptor blockers demonstrated a wide range of variability in clinical outcomes due to their unstable binding characteristics. As a result, prasugrel takes the place of clopidogrel utilizing its more predictable binding characteristics. The higher binding affinity of this drug increases the bleeding episode and is consequently enforced to treat individuals with a higher risk of acute coronary syndrome who are going through percutaneous coronary intervention (Stern & Lebowitz, 2010). To solve this acute problem, ticagrelor is being studied as the first reversible oral ADP antagonist, a specific inhibitor of the P2Y12 receptor on platelets, a crucial target receptor for ADP. A study reported that ticagrelor's safety and tolerability status corresponded to clopidogrel. These two drugs demonstrated the same significant bleeding profile, but a higher dose escalated the minor bleeding episode. As a reversible inhibitor of the P2Y12 receptor, ticagrelor facilitates several surgical procedures, including coronary bypass, after winding up the drug (Moons et al., 2003) . Vorapaxar or SCH 530348 is an antagonist of the protease-activated receptor (PAR)-1, the primary thrombin receptor on human platelet. Blockade of (PAR)-1 receptor inhibits thrombin-induced platelet aggregation without altering the functional activity of fibrinogen and thrombin. This drug is currently in phase III Thrombin Receptor Antagonist for Clinical Event Reduction (TRA*CER) trial to evaluate the safety and efficacy status for the treatment of acute coronary syndrome (Bonaca & Morrow, 2009; TRA•CER & The TRA•CER Executive and Steering Committees, 2009). Rivaroxaban and enoxaparin represent the direct oral inhibitor of factor Xa recommended against DVT and PE in the first instance and for managing recurrent DVT and PE. In patients with DVT or PE, rivaroxaban is safe and well-tolerated. Compared to enoxaparin-vitamin K antagonist, rivaroxaban was linked with a considerably lower rate of severe bleeding (Burness & Perry, 2014). Betrixaban (PRT054021) is another direct antagonist of factor Xa with better safety and efficacy than enoxaparin for the treatment of venous thromboembolism (VTE) after the replacement of the total knee. Currently, this drug is in Acute Medically III VTE (Venous Thromboembolism) Prevention with Extended Duration Betrixaban (APEX) trial to determine its safety and efficacy (Chan et al., 2014). American and European clinical practice guidelines emphasized bringing antiplatelet drugs into play for patients with cardiovascular diseases. Proper utilization of antiplatelet drugs can drop the mortality rate of CVD. This class of drugs, especially aspirin, is considered the primary prevention of CVD. This drug inhibits platelet aggregation by blocking the generation of TXA2 in platelet (by acetylation of COX in platelets) and blocks the prostaglandin synthesis in the vascular wall, which in turn causes vasorelaxation, preserves kidney function, and diminishes platelet adhesion to the vessel wall. Apart from antiplatelet activity, aspirin also exerts a direct effect on atheroma plaque in atherosclerosis patients (Chan, Hirsh, Ginsberg, & Eikelboom, 2014; Patrono et al., 1985; Tendera & Wojakowski, 2003). Antithrombotic and Thrombolytic Therapy: American College of Chest Physicians Evidence-Based Clinical Practice Guidelines (8th Edition) suggested the daily administration of low dose aspirin (75-100 mg) over no aspirin therapy, for the patients of 50 years or more without any symptomatic cardiovascular diseases. If maintained for ten years, aspirin brings down mortality modestly regardless of cardiovascular risk profile (Vandvik et al., 2012). These guidelines also recommended therapy for secondary prevention of patients with various diseases (Table 1). A diverse class of adjuvant treatments, including indirect antithrombin agents: heparin; direct thrombin inhibitors: hirudin, argatroban; antiplatelet agents: aspirin, dipyridamole, ticlopidine, clopidogrel, abciximab, tirofiban, and eptifibatide are used with thrombolytic agents (Table 2) (Baker, 2005). Again, heparin-induced thrombocytopenia (HIT) is one of the most important drug-induced clinical complications in hospitalized patients. HIT is an immune-mediated prothrombotic disease caused by therapy with unfractionated heparin (UFH) or low molecular weight heparin (LMWH). HIT is performed using antibodies that bind to macromolecular complexes formed by platelet autoprotein, platelet factor 4 (PF4), and heparin (Kelton, 2002). Agents used for adjuvant therapies are also present with a diverse class of adverse effects. Although the antithrombotic drugs possess potential side effects, including bleeding episodes, these drugs are widely used with adjuvant therapy to manage thrombus-induced CVDs.
Table 1
Therapeutic recommendation as secondary prevention of cardiovascular diseases for patients with various disease condition according to Antithrombotic and Thrombolytic Therapy: American College of Chest Physicians Evidence-Based Clinical Practice Guidelines (8th Edition).
Table 2
List of drugs for the treatment of thrombosis in cardiovascular disorder and their adverse effects.
Drug name | Mechanism of action | Dose with dosage regimen | Side effects | Toxicity | References |
Aspirin | Through irreversible acetylation of COX 1 Ser529 protein. | Adult dose: 75-300 mg daily Child dose: 60-100 mg orally. | Heartburn, bleeding, digestion complications. | Hyperventilation, dehydration, fever, double vision, and feeling faint. | |
Ticlopidine | Through irreversible suppression of P2Y12 receptor. | Adult dose: 250 mg two times daily for coronary artery stent thrombosis. | Bleeding, indigestion, heartburn, rash, neutropenia, TTP (thrombotic thrombocytopenic purpura). | Aplastic anaemia, agranulocytosis which can be accompanied by liver laceration. | |
Clopidogrel | Through irreversible suppression of P2Y12 receptor. | Adult dose: 75mg daily orally. | Bleeding, rash, neutropenia and in rare case TTP. | Prostration, difficult breathing, and gastrointestinal haemorrhage in animals. | (Balamuthusamy & Arora, 2007; Michelson, 2008; Quinn & Fitzgerald, 1999) |
Prasugrel | The active metabolite irreversibly inhibits the P2Y12 receptor | Adult dose: 10 mg daily orally. | Heartburn, indigestion, nausea, vomiting. | Gastrointestinal bleeding and thrombocytopenia. | (Jakubowski, Winters, Naganuma, & Wallentin, 2007; Nanau, Delzor, & Neuman, 2014) |
Abciximab | Integrin αIIbβ3 antagonist | Adult dose: injection solution 2mg/ml 0.125 µg/kg/min once via IV for unstable angina. | Heartburn, indigestion, nausea, vomiting. | Gastrointestinal bleeding and thrombocytopenia | |
Eptifibatide | Integrin αIIbβ3 antagonist. | Adult dose: injection solution 2 mg/ml or 0.75 mg/ml once (180µg/kg) via IV for the acute coronary syndrome. | Bleeding, pseudo thrombocytopenia, hypotension. | Renal insufficiency, increase the risk of bleeding and heart failure. | |
Dipyridamole | Through the inhibition of adenosine uptake and cyclic nucleotide phosphodiesterase. | The adult dose: 75-100 mg two or three times orally. | Hypotension and inconsistent blood pressure, dizziness, flushing, diarrhea and abdominal discomfort, headache, rash etc. | Increases the risk of bleeding. | (Henzlova, Duvall, Einstein, Travin, & Verberne, 2016; Lee, Di, Huang, Peng, & Hu, 2017; Lee, Ku, Kim, & Bae, 2017; Patrono, Baigent, Hirsh, & Roth, 2008) |
Cilostazol | Through the inhibition of adenosine uptake and cyclic nucleotide phosphodiesterase 3. | The adult dose: 100 mg twice daily orally. | Bleeding, headache, diarrhea, palpitations, dizziness, rash, pancytopenia | Severe hypotension, tachycardia, and possibly cardiac arrhythmia | (Ahmad, Degerman, & Manganiello, 2012; Rogers, Oliphant, & Finks, 2015) |
Warfarin | Abolish the synthesis of active clotting factors through the inhibition of VKORC1 (vitamin K epoxide reductase complex 1). | Depends on guidelines; usually 5 mg per day. | Haemorrhage and bleeding, abdominal discomforts, flatulence, bloating, skin necrosis and altered sense of taste. | Increases the risk of bleeding. | (Kuruvilla & Gurk-Turner, 2001; Teles, Fukuda, & Feder, 2012) |
Rivaroxaban | Inhibits Factor Xa through reversible and direct binding with Factor Xa via the S1 and S4 pockets. | Adult dose: 10/20 mg orally once daily. | Haemorrhage, dizziness, insomnia, fatigue, depression, anxiety, abdominal pain. | Bleeding episode. | |
Heparin | Inhibits the FXa and thrombin in the coagulation cascade, thereby suppress the transformation of fibrinogen to fibrin. | Adult dose: 5,000-10,000 units every 4-6 hours (intravenous injection) | Bleeding, thrombocytopenia. Chronic use of aspirin generates osteoporosis and osteopenia. | Severe bleeding episodes. | |
Ticagrelor | Through antagonism of P2Y12 receptor. | 50 mg twice/day, 100 mg twice/day, 200 mg twice/day, or 400 mg/day | Bleeding, dyspnea, increase in ventricular pauses, | Risk of severe bleeding. |
Pathways to understanding the antithrombotic effects of natural compounds from both plant and animal sources
From ancient times, human civilization has been reclined on the medicinal plant to alleviate major and minor ailments; medicinal plants and plant-derived bioactive compounds have always been suitable for treating various communicable and non-communicable diseases. Since ancient times, people have primarily dependent on natural heals to cure various communicable and non-communicable diseases (Shrivastava et al., 2022). It is well established that the pharmacological activity of natural medicine is attributed to the presence of various bioactive compounds, including phenolics, flavonoids, polysaccharides, proteins, peptides, terpenes, alkaloids, and several others. Multiple lines of evidence demonstrated that plant-derived bioactive compounds gradually became the first treatment choice for impeding thrombus formation and thrombus-induced cardiovascular and cerebrovascular diseases (Shaito et al., 2020). Phenolics or phenolic compounds are recognized as secondary metabolites ubiquitously distributed in various plant species. These compounds have the same chemical structure: an aromatic ring with one or more -OH (hydroxyl) substituents. Phenolic compounds can be classified into several groups, including flavonoids, tannins, stilbenes, lignans, and phenolic acids. These compounds are most prominently distributed in medicinal plants and exert various pharmacological roles (Alu’datt et al., 2017). Flavonoids represent the largest group of phenolic compounds, composed of fifteen carbon atoms in order in three rings (C6–C3–C6) marked as A, B, and C, respectively. Flavonoids can be subdivided into various groups, including flavones, flavonols, dihydrochalcones, isoflavones, aurones, flavanones, flavanones, chalcones, flavans, anthocyanins, and proanthocyanidins based on C-ring substituents and degree of saturation (Xu, Wang, Pu, Tao, & Zhang, 2017). On the other hand, terpenes or isoprenoids represent the single largest group of essential oils, consisting of isoprene molecules where each molecule contains five carbon atoms with double bonds. Based on the number of isoprene molecules, terpenes can be classified as monoterpenes (2 isoprene molecules), sesquiterpenes (3 isoprene molecules), and diterpenes (4 isoprene molecules) (Hanuš & Hod, 2020). Alkaloids are cyclic organic compounds with heterocyclic tertiary nitrogen in the structure. But caffeine, paclitaxel, and colchicine are the exception to this definition. The molecular skeleton can classify alkaloids into various vital groups, including indole alkaloids, isoquinoline alkaloids, tropane alkaloids, steroidal alkaloids, and pyridine and pyrrolizidine alkaloids. Multiple studies proved that alkaloids isolated from medicinal plants demonstrated antiplatelet activity in different experimental models (Ain, Khan, Mubarak, & Pervaiz, 2016).
Agents against thrombus formation exert their activity through multiple mechanisms, including inhibiting platelet aggregation and thrombus formation (antiplatelet agents), preventing the coagulation system, and interfering with further plaque expansion (anticoagulants). Natural phenolics, including polyphenols and flavonoids, have demonstrated effectiveness as a preventive and alternative medicine to manage thrombus-induced cardio and cerebrovascular complications. They are reported to exhibit antiplatelet and antithrombotic action via the reduction of oxidative stress, (Ciumărnean et al., 2020; Freedman, 2008) decreases intracellular Ca2+ mobilization,(Lutz et al., 2019) inhibit platelet phospholipase C,(Hsiao et al., 2005) ameliorate intraplatelet cyclic adenosine monophosphate (cAMP) level,(Oh et al., 2012) antagonize TXA2 receptor, (Guerrero et al., 2005) and antagonize thrombin receptor (Navarro-Núñez et al., 2008).
The generation of ROS and RNS (reactive nitrogen species) within the body promotes oxidative stress, generating various physiological consequences, including platelet hyperactivation and risk of thrombus formation. It is well established that phenolics have tremendous potential to scavenge free radicals and act as a potent antioxidants (Santhakumar, Bulmer, & Singh, 2014). For instance, ascorbate or vitamin C is the best example of an antioxidant that possesses tremendous capability in scavenging ROS and reducing oxLDL (oxidized LDL) cholesterol in atherosclerotic conditions (Aguirre & May, 2008). Cinnamtannin B-1 is a proanthocyanidin isolated from bay wood that has been reported to reserve the generation of oxidants and reduce platelet aggregation in type-2 diabetic models (Bouaziz et al., 2007). Olive leaves and olive oil polyphenols demonstrate tremendous antioxidant activity through activating phospholipase C and promoting the metabolism of arachidonic acid (AA), reducing H2O2 levels. These events not only prolong atherosclerosis but also inhibit platelet aggregation (Singh, Mok, Christensen, Turner, & Hawley, 2008). Grape seed extract and resveratrol (polyphenol in grape) were reported to reduce the blood platelet function by decreasing P-selectin expression, inhibiting platelet signaling pathways, and decreasing the production of superoxide anions in blood platelet (Olas, Wachowicz, Stochmal, & Oleszek, 2012). Therefore, quotidian consumption of phenolic-rich foods might protect against CVDs and antithrombotic action by inhibiting platelet hyperactivation or aggregation. Platelets release a spike of H2O2 in response to collagen stimulation, which stimulates phospholipase C and AA metabolism. Stimulation of AA promotes the release of prostaglandin H2 (PGH2) and TXA2, which are thought to activate the platelet intracellular signaling pathway and play a significant role in platelet activation (Figure 5). Polyphenolic antioxidants target H2O2 to neutralize this pernicious free radical and put a pivotal role in impeding platelet activation (Pignatelli, Pulcinelli, Lenti, Gazzaniga, & Violi, 1998; Santhakumar et al., 2014). Free radicals scavenging capacity of polyphenols impart numerous beneficial effects within the body, and it has been reported that a polyphenolic-rich diet vastly reduces cardiovascular mortality and thrombotic diseases (Massimo, Scarpelli, Penco, & Tozzi-Ciancarelli, 2004). (Carnevale et al., 2012) reported that in smokers, polyphenols-rich dark chocolate down-regulates platelet ROS production, Nox2 (NADPH oxidase) activation, inhibits platelet activity by reducing the formation of platelet 8-ISO-prostaglandin F2α. Platelet 8-ISO-prostaglandin F2α is a bioactive product of AA peroxidation, responsible for enhancing platelet response to agonists through the stimulation of glycoprotein IIb-IIIa (GP IIb-IIIa) (Carnevale et al., 2012).
Figure 5
Dietary polyphenols inhibit oxidative stress-induced platelet aggregation through impeding H2O2 generation and activation of platelet intracellular signaling. AA= arachidonic acid; PGH2= prostaglandin H2; TXA2= thromboxane A2; COX1= cyclooxygenase 1; PAR= protease-activated receptor; VWF= von Willebrand factor.
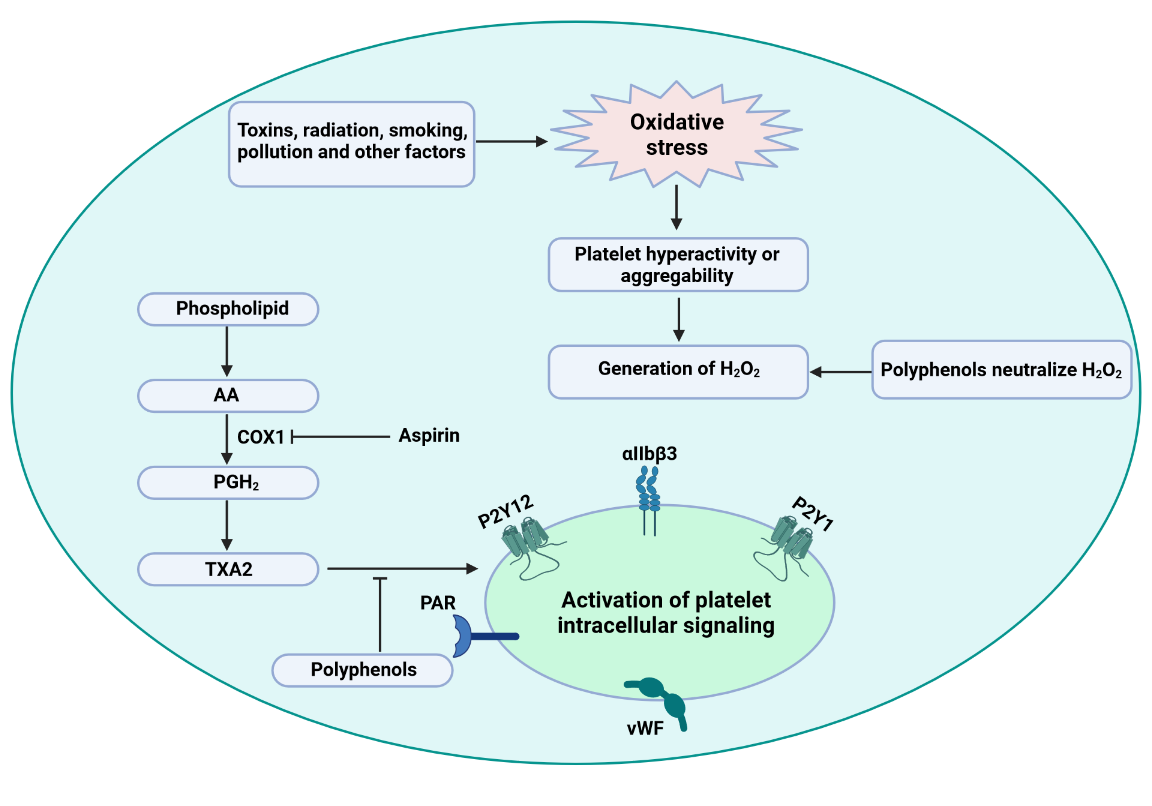
An increase in platelet activation dramatically raises intracellular Ca2+ to near macromolecular level and promotes the release of phospholipases Cβ and Cγ, which directly control the Ca2+ mobilization into the cytosol (Fuentes & Palomo, 2014). Various phenolic compounds, including rutin, α-naphthoflavone, obovatol, and rutaecarpine, downregulate AA. Serotonin liberation, phosphorylation of P47, and intracellular Ca2+ mobilization through the inhibition of phospholipase C activity, PKC (protein kinase C) activation, and TXA2 formation, therefore produce antiplatelet activity (Hsiao et al., 2005; Park et al., 2011; Sheu, Hsiao, Chou, Shen, & Chou, 2004). cAMP downregulates intracellular Ca2+ level in human platelet through the cAMP-dependent protein kinase a (PKA), which is involved in the inhibition of cytoskeletal reorganization (Rosado, Porras, Conde, & Sage, 2001). cAMP exerts a modulatory role over Phospholipase C mediated secretion and human platelet aggregation Huzoor-Akbar, Navran, Chang, Miller, and Feller (1982). Epigallocatechin gallate (EGCG), a polyphenolic compound, raises cAMP levels in the collagen-platelet interaction, potentially preventing platelet activity and aggregation (Ok et al., 2012). Previously, we mentioned that TXA2 is produced as a sequential result of AA metabolism. Upon activation with the help of agonists like ADP, thrombin, and collagen, TXA2 acts as a potent unstable inducer of platelet aggregation and activation. After vascular damage, TXA2 recruits and activates surrounding platelets of the vascular damage site (Arita, Nakano, & Hanasaki, 1989). So, inhibition of the TXA2 receptor can be an excellent therapeutic approach against thrombus-induced physiological consequences. A diverse class of flavonoids says genistein, apigenin, and luteolin inhibit collagen and ADP-induced platelet activation; reduce the extent of TXA2 secretion in collagen and ADP-stimulated platelets through binding with TXA2 receptor. It can be simplified that genistein, apigenin, and luteolin antagonize TXA2 receptors and inhibit platelet aggregation and activation (Guerrero et al., 2005). Berberine and clausine-D alkaloids were reported to produce antiplatelet action through inhibiting ADP, AA, and collagen-induced TXA2 generation (Ain et al., 2016). Thrombin is an enzyme derived from the family of serine protease, known as active plasma coagulation factor II. It is a critical enzyme that regulates the second phase of the blood coagulation cascade by generating TF-bearing cells (responsible for blood platelet activation) and stimulating other plasma coagulation factors, including FXI and FVIII FV on the platelet's surface. Recently, a study conducted on eleven isoquinoline alkaloids revealed seven of them: jatrorrhizine, 8-trichloromethyl-dihydroberberine, berberine, acetonyl-berberine, palmatine, L tetrahydropalmatine, and L-corydalmine significantly inhibited the activity of TF. Further, berberine, L-corydalmine, L tetrahydropalmatine, and jatrorrhizine also stably bonded with the active site of the TF/FVIIa complex in the molecular docking study, which validates their potentiality in the treatment of cardiovascular diseases (He, Zeng, & Jiang, 2021). So, agents that can inhibit thrombin from binding to thrombin receptors can be a lead compound for developing antithrombotic drug therapy. Various phenolics like silybin, cyanidin, (+) catechin quercetin, cyanin, and (-) epicatechin can directly inhibit thrombin amidolytic activity or change the proteolytic activity of thrombin (Bijak et al., 2014).
Plant-derived proteins, peptides, and polysaccharides produce antithrombic effects through several mechanisms. Peptides from a stable thrombin-antithrombin complex and the active site of peptide slow down the thrombin and fibrinogen activity, where fibrinogen is transformed into fiber protein to achieve the antithrombotic activity. It has been reported that three protein fractions (water-, salt- and acid-soluble) of Mytilus edulis showed antithrombotic efficacy in both in vitro and in-silico studies (Figure 6) (Qiao et al., 2018). Polysaccharides have been described as the most therapeutically investigated metabolites, and isolation of polysaccharides from natural sources may become an excellent approach for developing new and orally effective antithrombotic therapies; piquing the interest of researchers looking to develop new antithrombotic therapies from natural polysaccharides. It has been reported that polysaccharides from natural sources, especially marine-derived polysaccharides, demonstrate tremendous potential through several mechanisms, including; enhancement of thrombin time (TT) and activated partial thromboplastin time (APTT), reduction of PAI-1 level, inhibition of thrombin and FXa activity, inhibition of thrombus formation, and inhibition of platelet aggregation (Figure 6). Prolongation of APTT occurs due to the scarcity of factors VIII, IX, XI, XII, and V or Willebrand's factors. On the other hand, prolonging prothrombin time (PT) represents the extrinsic coagulation pathway and occurs due to the lack of coagulation factors like V, VII, and X. However, polysaccharides isolated from various species of sea cucumber including Cucumaria frondosa, Cucumaria japonica, and Massinium magnum, brown algae including Sargassum aquifolium, and Hormophysa cuneiformis; sea urchin including Echinometra lucunter, and Strongylocentrotus franciscanus, showed antithrombotic activity in the various experimental setup (Carvalhal et al., 2019).
Apart from medicinal plants, animal sources like snake venoms, leeches, and bats' saliva are also enriched with antithrombotic compounds, which proved their potentiality in the various experimental set up with similar mechanisms to other antithrombotic therapeutics (Figure 6). Species of leeches cover most animal-derived antithrombotic bioactive compounds, and some of them are under investigation as pharmaceutical drugs. For instance, decorsin is a polypeptide isolated from the Macrobdella decora leech, acting as a platelet aggression inhibitor by potentially blocking the activity of glycoprotein IIb-IIIa (Table 3). This leech-derived peptide is under the investigation of Genentech, South San Francisco, California, the United States, as a pharmaceutical drug (Sawyer, 1991).
Table 3
List of antithrombotic natural compounds isolated from various animal sources with their possible mechanisms.
Name | Source | Anti-thrombotic mechanism | References |
Lufaxin | Saliva of Lutzomyia longipalpis fly | Inhibitor of factor Xa (FXa). Stop up prothrombinase. Enhance PT and APT. | |
Tick anticoagulant peptide | Ornithodoros moubata soft tick extract | Produce anticoagulant effect through inhibiting factor Xa. | |
Hirudin, Antistasin, Ghilanten | Leeches | Produce anticoagulant effect through inhibiting factor Xa. | |
Decorsin | Leech (Macrobdella decora) | Inhibit platelet aggression through antagonizing glycoprotein IIb/IIIa. | |
Anticoagulant peptide | Ancylostoma caninum | Inhibit factor Xa and produce anticoagulation effect. | |
Haemadin Thromin | Haemadipsa sylvestri, Theromyzon tessulatum | Generate anticoagulation through inhibiting thrombin. | (Salzet, Chopin, Baert, Matias, & Malecha, 2000; Strube, Kroger, Bialojan, Otte, & Dodt, 1993) |
Dipetalogastin | Dipetalogaster maximus | Inhibit thrombin and extend cloting time. | |
Rhodniin Infestin Brasiliensin | Rhodnius prolixus, Triatoma infestans, Triatoma brasiliensis | Thrombin specific anticoagulation effect. | (Araujo et al., 2007; Campos et al., 2002; Friedrich et al., 1993) |
Peptides | Glossina morsitans morsitans | Suppress tsetse thrombin. | |
Thrombostasin Savignin Amblin | Haematobia irritans, Ornithodoros savignyi, Amblyomma hebraeum | Inhibit thrombin induced blood coagulation. | (Lai, Takeuchi, Jonczy, Rees, & Turner, 2004; Nienaber, Gaspar, & Neitz, 1999; Zhang, Cupp, & Cupp, 2002) |
Proteins | Boophilus microplus | Inhibit thrombin induced platelet aggregation. Inhibit both intrinsic and extrinsic coagulation pathways. | |
Bothrojaracin | Bothrops jararaca | Suppress the secretion and aggregation of platelet. | |
ACH-11 peptide | Agkistrodon acutus Venom | Suppress ADP induced platelet aggregation. Block the catalytic role of FXa |
Figure 6
Antithrombotic mechanisms of various bioactive natural compounds from both plant and animal sources. cAMP= cyclic adenosine monophosphate; TXA2= thromboxane A2; OS= oxidative stress; TF= tissue factor; TT= thrombin time; APTT= activated partial thromboplastin time; PAI-1= plasminogen activator inhibitor-1;FXa= factor Xa.
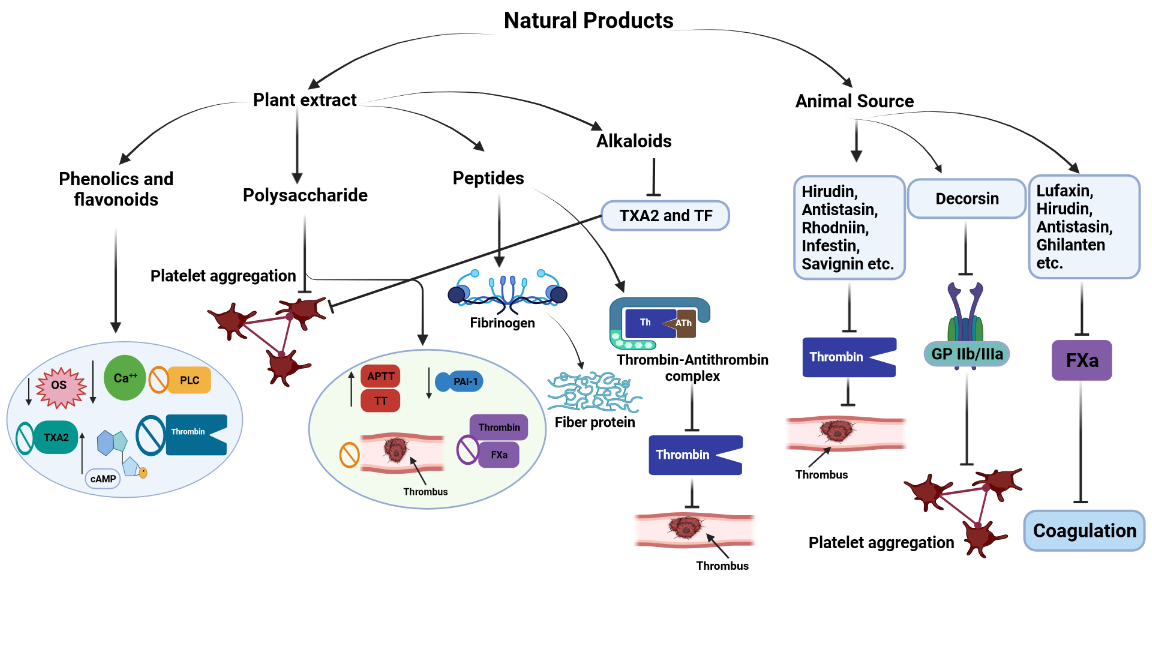
In vitro and in vivo antithrombotic investigation of different plant extracts
Research is still searching for safe compounds to treat thrombus-induced cardiovascular and cerebrovascular disorders. Medicinal plants extract from diverse families, and their active metabolites gradually become potential candidates for this purpose. They have been reported to produce a significant antithrombotic effect in various experimental models or produce a synergistic impact without major episodes of adverse effects when administered in combination with traditional drugs. For instance, Rhus verniciflua Stokes belongs to the family of Anacardiaceae and is well recognized for its antiplatelet activity. The active compounds (Fisetin, Butein, and Sulfuretin) were isolated from the bark of this auspicious plant; among them, Fisetin inhibited platelet aggregation by preventing collagen-induced ERK (extracellular signal-regulated kinase) and MAPK (mitogen-activated protein kinase) activation (Lee et al., 2014). Ginkgo biloba L. under the family Ginkgoaceae is one of the most widely used commercial medicinal plants, various parts of this plant have been included in ancient herbal medicine.
Flavonoid glycosides (kaempferol, quercetin, and isorhamnetin), biflavones, terpenoids (ginkgolides and bilobalides), and various organic acids are the active phytoconstituents of this plant which are strongly involved in various pharmacological activities (Shaito et al., 2020). Studies reported that different doses of G. biloba L. extract could potentiate the effect of cilostazol; a combination of these two can more efficiently inhibit platelet aggregation without increasing side effects (Ryu et al., 2009). Polyphenols, monoterpenes, flavones, flavonoids, and polyphenolic polysaccharide conjugates are the most active constituents of the medicinal plants belonging to various families. Crataegus pinnatifida Bge (Hawthorn), Rubus chingii Hu, Chaenomeles sinensis (Thunb.) Koehne, Filipendula ulmaria L., Rubus plicatus W. et N. Fragaria vesca L., are several major species of the Rosaceae family showing antithrombotic activity by various mechanisms.
Kaempferol, quercetin, polysaccharides, and polyphenolics are the leading active players responsible for platelet aggregation or anticoagulation activity (Han et al., 2012; Pawlaczyk, Czerchawski, Pilecki, Lamer-Zarawska, & Gancarz, 2009). Asteraceae is another affluent plant family with antithrombotic activity. It has been reported that Erigeron canadensis L. is a rich source of polyphenolic-polysaccharide conjugates, which produce antiplatelet and anticoagulant effects through AA-induced cyclooxygenase pathway as well as by inhibiting thrombin and factor Xa activation (Pawlaczyk et al., 2011). Flowering parts of Solidago virgaurea L., Echinacea purpurea L. Moench. and Arnica montana L., are exuberant with various polysaccharides, monosaccharides (Rha, Ara, Man, Glc, Gal), and polyphenolics. These plants have been reported to completely inhibit plasma clot formation in APTT study models at a low concentration, proving the anticoagulation evidence in favor of these plants (Pawlaczyk et al., 2009). Leonotis leonurus L. from the Lamiaceae family and its isolated active compound Marrubiin showed anticoagulant action by increasing APTT and suppressing platelet aggregation, inflammatory markers, and coagulation markers in various in vitro and in vivo study models. Both plant extract and its active compound dose-dependently reduce protein secretion, intracellular Ca2+ mobilization, platelet adhesion, fibrin formation, and inhibit thromboxane B2 (TXB2) generation (Mnonopi, Levendal, Davies-Coleman, & Frost, 2011). Active compounds of Thymus vulgaris L. and three different extracts of various parts of Salvia deserta Schang produced antiplatelet aggregation effects in the different experimental setups (Kasimu et al., 2018; Okazaki, Kawazoe, & Takaishi, 2002). Numerous medicinal plants and plant-derived bioactive compounds derived from Poaceae, Cucurbitaceae, Araliaceae, Euphorbiaceae, Apiaceae, and Piperaceae families have also been reported to produce thrombus-induced cardioprotective activity by various mechanisms (Emon et al., 2021; Félix-Silva et al., 2014; Gadi et al., 2009; Kim et al., 2008; Li, Wang, & Xu, 2013; Osoniyi & Onajobi, 2003; Rajput, Balekar, & Jain, 2014; Song, Yang, Yu, & Li, 2011; Yogeswari, Bindu, Kamalraj, Ashokkumar, & Jayabaskaran, 2020). Table 4 and Table 5 represent the antithrombotic activity of different plant extracts in the experiment set up with their possible mechanism of action.
Table 4
In vitro investigation of plants and natural medicinal compounds extract for antithrombotic effects.
Table 5
In vivo investigation of plants and natural medicinal compounds extract for antithrombotic effects.
Table 6
Bioactive compounds that show in vitro antithrombotic activity.
Compound name | Compound class | Source | Dose/ conc. used | Experimental setup | Dose/conc. showed the significant effect | Possible mechanism of action | References |
α-Naphthoflavone (α-NF) | Prototype flavone | Common in diet. | 5-20 µM | Human platelet suspensions were prepared and platelet aggregation was measured by turbidimetric method. | 5 and 10 µM doses inhibited platelet aggregation. | Inhibit the activation of phospholipase C, which leads to the inhibition of intracellular Ca2+ mobilization. Activate the formation of cyclic GMP which in consequence suppress platelet aggregation. | |
Rutin | Bioflavonoid | Common in diet. | 250 and 290 µM | Human platelet aggregation was measured by turbidimetric method. | 290 µM dose inhibited collagen induced platelet aggregation. | Inhibit Ca2+ mobilization which in consequence suppress platelet aggregation. Impede TXA2 formation. | (Sheu et al., 2004) (Sheu et al., 2004) |
Epigallocatechin Gallate | Flavonoid | Green tea | 25–200 µM | Platelet rich plasma was prepared from the blood of rabbit and platelet aggregation was determined. | 200 µM dose inhibited collagen induced platelet aggregation. | Block phosphorylation of collagen mediated protein tyrosine and phospholipase (PL) Cγ2. Decrease serotonin secretion, cytosolic calcium mobilization and AA liberation. Block Ca2+–ATPase inhibitor. Elevate AA-mediated PGD2 formation. | |
Quercetin, Apigenin and Catechin | Flavonoid, Flavone and Phenolic compound respectively. | Dietary source | Different concentrations. | Human platelets were prepared to conduct aggregation and 5-HT secretion experiments. | Apigenin at 10 µM and 40 µM doses inhibit platelet aggregation. Quercetin at 40 µM dose completely abolish aggregation. They also inhibited 5-HT secretion. | Impede platelet aggregation and 5-HT release. Inhibit the phosphorylation of total protein, PLCγ2 tyrosine and Syk. Inhibit Fyn kinase activity. | |
Quercetin | Flavonoid | Fruits, vegetable, red wine, and tea. | 12.5–100 μM | Blood was retrieved from the abdominal artery of 8∼10 weeks old rats and platelet aggregation was determined. | 25 μM inhibits platelet aggregation by 50%. | Suppress platelet aggregation. Inhibit collagen-induced P-selectin expression, [Ca2+] i mobilization, ATP release and integrin-αIIbβ3 activation. Ameliorate cAMP and vasodilator-stimulated phosphoprotein (VASP) levels. Inhibit platelet aggregation by reducing p38 MAPK, PI3K, Akt, JNK1 and ERK2 activations. | |
Genistein | Phytoestrognic isoflavone |
| 1-30 μg/mL | Human platelet suspension was prepared and platelet aggregation was measured. | 10 μg/mL dose inhibited U466lP induced platelet aggregation and serotonin release. | Inhibit tyrosine kinases. Inhibit serotonin secretion. Inhibit phospholipase C activity. Inhibit protein kinase C activity. | |
Equol | Soy isoflavones | Soy-based foodstuffs. | 5, 10, 30 μmol/L | Human washed platelet was prepared and platelet aggregation was evaluated. | Produced dose dependent platelet aggregation. | Antagonize thromboxane A2 receptor. | |
Orientin and isoorientin | C‐glycosidic flavonoids | Vaccinium bracteatm Thunb. | Different doses | Washed human platelets were prepared, platelet aggregation and APTT and PT was measured. | Orientin and isoorientin at 5 and 20 μM dose prolonged APTT and PT. They inhibited thrombin and U46619 induced platelet aggregation in concentration dependent manner. | Impede platelet aggregation and thrombin-catalyzed fibrin polymerization. Suppress calcium mobilization and PKC activation. Decrease the PAI-1 to t-PA ratio. | |
Pelargonidin | Anthocyanidin | Found as red pigment in plants. | 0, 2, 5, 10, 20, 30 μM | Human platelets from healthy volunteers were prepared and platelet aggregation and APTT and PT was measured. | 10 μM or higher dose prolonged APTT and PT. Pelargonidin inhibited reptilase and U46619 induced platelet aggregation in concentration dependent manner. | Decrease fibrin polymerization. Decrease human platelet aggregation. Decrease the amidolytic activity of thrombin. Inhibit the activation and production of FX and thrombin. Abolish the ration of PAI-to t-PA. | |
Scolymoside | Flavonoid | Cyclopia subternata | 0-30 μM | Washed platelets from syngeneic donor mice and platelet aggregation was determined. | 29.4 μM (IC50 for inhibition of platelet aggregation). | Impede platelet aggregation and thrombin-catalyzed fibrin polymerization. Abolish the ration of PAI-to t-PA. | |
Zingerone | A phenolic alkanone | Zingiber officinale Roscoe. | 1, 5, 10, 25, 50 μM | Purified human PRP was prepared and platelet aggregation was determined by aggregometer. | 19.94 μM doubled the clotting time. Suppressed platelet aggregation in a dose dependent manner. | Inhibit the catalytic activity of FXa. Inhibit phosphorylation of MARCKS (myristoylated alanine-rich C-kinase substrate), platelet P-selectin and PAC-1 expression. | |
Cyclo(L-Pro-L-Tyr) and N-acetyltyramine | Diketopiperazine and Phenylethanoid respectively | Tenebrio molitor (insect) | 1, 5, and 10 μM | Clotting time was measured from mice blood and platelet aggregation was determined from human PRP. | At 2.43, and 3.40 µM respectively doubled the clotting time and both inhibited platelet aggregation in a concentration dependent manner. | Impede FXa and platelet aggregation activities. Prolongs APTT. | |
Pinnatifidanoside F | Sesquiterpenoid | Crataegus pinnatifida Bunge | 0.25 mg/mL | PRP was prepared from rat and platelet aggregation activity was measured. | 0.25 mg/mL concentration inhibited platelet aggregation by 84%. | Impede ADP induced platelet aggregation. Increase the time to form thrombocytes. | |
Geniposide | Iridoid glycoside | Gardenia jasminoides Ellis | 2.6, 7.7, 26 and 77 mM | Platelet aggregation was measured by 4 channel aggregometer. | Geniposide inhibited collagen induced platelet aggregation by 84%. | Inhibit collagen-induced platelet aggregation. Inhibit phospholipase A2 (PLA2) activity. | |
Nuzhennoside and Nuzhennoside G13 | Secoiridoid glucosides | Osmanthus fragrans Lour. | 0.7, 1.4 and 2.8 mg/kg | Platelet aggregation was measured from rabbit PRP. | Nuzhennoside G13 at 2.8 mg/kg showed greatest inhibition of platelet aggregation. | Inhibit ADP and collagen induced platelet aggregation. | |
Ambinine | Alkaloid | Corydalis ambigua var. | 0.5, 1 or 2 mg/mL | Plasma recalcification time (PRT) and blood clot assay was performed with fresh rat blood. | 2 mg/mL dose prolonged PRT and degraded the blood clot. | Prolong PRT. Degrade blood clot through interfering with intrinsic coagulation cascade. | |
Ferulic acid | Hydroxycinnamic acid | Various medicinal plants. | Different doses for different model | In vitro anticoagulation and aggregation study was performed with rat platelet. | 200 μM dose inhibited thrombin, ADP, U46619 and collagen induced platelet aggregation. | Inhibit platelet aggregation. Reduce intracellular Ca2+ mobilization and TXB2 production. Increase cAMP level. | |
Epi-sesamin | Lignan | Asarum sieboldii | 0.5, 1, 2, 5, 10 and 20 μM | Anticoagulation study was performed with citrated normal human plasma and platelet aggregation assay was performed with mouse PRP. | Greater than 2 μM dose significantly prolonged APTT and PT. Inhibited platelet aggregation in concentration dependent manner. | Impede the functions and production of FXa and thrombin. Impede platelet aggregation fibrin polymerization. Decrease the PAI-1 to t-PA ratio. |
Table 7
Bioactive compounds that show in vivo antithrombotic activity.
Table 8
Clinical trial of antithrombotic natural compounds from both plant and animal source to investigate their safety and efficacy.
In vitro and in vivo screening of probable active compounds having the antithrombotic effect
Plant-derived active metabolites gradually become the main target of a safe agent that can satisfy clinical outcomes with little or no side effects. They already have proven significant antithrombotic effects in previous in vitro and in vivo setups. Hence would be a probable solution to treat cardiovascular disorders alone or in combination with current therapy to produce a synergistic effect without any chance of having severe adverse reactions. In this part, we have listed the bioactive compounds studied in different experimental sets to explore their possibility of becoming effective for better managing thrombotic episodes in cardiovascular disorders (Table 6 and Table 7).
Current use of natural antithrombotic compounds and compounds in the clinical trial
Gradually natural bioactive compounds are becoming mainstream to treat thrombus-induced cardiovascular and cerebrovascular diseases. For example, Danhong injection (DHI) is one of the most prescribed Chinese medicine injections, prepared from the aqueous extracts of two widely used Chinese medicinal plants named Salvia miltiorrhiza Bunge and Carthamus tinctorius L., which generate synergistic therapeutic effects due to their multi-components and multi-targets. A bunch of seven salvianolic acids are the primary ingredients of this injection and have a remarkable impact on managing pernicious diseases, including cardiovascular and cerebrovascular complications in Chaina. It has been reported that the combination of salvianolic acids A and C synergistically inhibit platelet aggregation in vitro experimental models (Zhao et al., 2017). Guanxinning Tablet is another modern Chinese drug made from two traditional Chinese medicinal herbs named Ligusticum striatum DC and Salvia miltiorrhiza Bunge., which are reported to produce a synergistic effect in improving blood circulation and suppressing thrombus formation. Senkyunolide I and cryptotanshinone, two bioactive compounds isolated from these herbs, also produce a synergistic antithrombotic effect (Li et al., 2021). Administration of Ginkgo biloba extract in combination with cilostazol has been reported to enhance the antithrombotic activity of cilostazol without any episode of adverse impacts (Ryu et al., 2009). In another study, it has been found that Campomanesia xanthocarpa extract produces a synergistic effect when administered in combination with acetylsalicylic acid and increases the potentiality of acetylsalicylic acid to inhibit platelet aggregation (Otero et al., 2017). Natural bioactive compounds can be considered medicinal agents for treating cardiovascular and cerebrovascular complications with pathological clotting.
Apart from administration with other drugs, various antithrombotic therapy derived from natural sources (plants and animals) were tested in clinical trials to investigate their safety and efficacy. Although the number of attempts at natural antithrombotic compounds is still not significant, initiatives are gradually taken to insert natural compounds into human trials (Li et al., 2021; Wang et al., 2015). The more substantial number of tests will be conducted, more opportunities will create to discover safer antithrombotic therapies. Table 8 demonstrates the outcome of clinical trials conducted on natural compounds from plant and animal sources to determine their safety and efficacy in the human model.
It is well known that currently available antithrombotic drugs have substantial side effects (i.e., internal hemorrhages), which demand more extensive research to discover a safe and efficient one. Because of their ability to preserve normal hemostasis while lowering internal bleeding and avoiding unexpected clot formation, the mechanism of action of antiplatelet and anticoagulant natural compounds has been the research focus. However, little has been known regarding how therapies from natural products possess lower risk than traditional therapy. But human trials on several natural compounds revealed their safe therapeutic profile. For instance, desirudin is an analog of hirudin, when administered subcutaneously, produces direct thrombin inhibitory effect without significant bleeding episodes. In 1997 European medicines agency approved the administration of desirudin to manage DVT in patients’ surgery like total knee replacement or total hip replacement. So extensive research is required to discover the novel mechanisms of natural antithrombotic compounds regarding how they are comparatively safer than traditional therapy.
Safety of natural antithrombotic drugs in context of current therapy
Due to the life-threatening bleeding risk of conventional antithrombotic drugs, researchers are now inclined to discover safer therapy from natural sources. Therapies target various receptors, majorly COX1, P2Y12, ADP, thrombin, thromboxane, integrin αIIbβ3, cAMP, calcium, VKORC1, PDE, prostacyclin, and prostaglandin to produce antiplatelet and anticoagulant action (Zhang, Li, Ye, Chen, & Chen, 2021) . Most antithrombotic drugs are multitargeted and non-specific drugs. For instance, low molecular weight heparin and unfractionated heparin work on several coagulation factors. Combination therapy for the management of thrombus-induced cardiovascular diseases may potentiate the risk of bleeding. Although the reduction of the bleeding mechanism by natural antithrombotic therapies is still not apparent, these agents may target specific coagulation factors. Rigorous research is necessary to uncover their exact mechanism.
Conclusion and future direction
In developed countries, the increasing pattern of sedentary lifestyles is responsible for developing various pernicious diseases, including CVDs and thrombosis, recognized as the primary cause of global death and disability. The death rate from these diseases is increasing alarmingly, and researchers are still working onerously to uncover the possible solution to this emergency, including the development of complementary therapeutic agents, search for natural bioactive compounds with maximum therapeutic efficacy and minimal adverse effects, formulation of functional foods to increase blood circulation and finally changes in lifestyle pattern. Isolation of bioactive compounds from natural sources with antithrombotic activity might produce promising alternatives to current treatments and replace the conventional therapies with potentially adverse effects. As the therapy has been recognized for its auspicious role in thrombotic disorders, more research should be conducted to isolate potential compounds and drive them in human trials to determine their safety and efficacy. Administration of these compounds with traditional drugs can also improve the therapeutic efficacy of the drug. Therefore, continuous investigation of natural products with antithrombotic properties can yield new insights into new drugs with better safety and efficacy.
Abbreviations
5HT, 5-hydroxytryptamine receptors; AA, arachidonic acid; ADP, adenosine diphosphate; APTT- activated partial thromboplastin time; cAMP, cyclic adenosine monophosphate; CVDs, cardiovascular diseases; ERK, extracellular-signal-regulated kinase; ICAM, intercellular adhesion molecule; IHD, ischemic heart disease; JNK, c-Jun N-terminal kinase; HMWK- high molecular weight kininogen; MAPK, mitogen-activated protein kinases; PGH2, prostaglandin H2; PGI2, prostaglandin I2; PT, prothrombin time; ROS, reactive oxygen species; TF, tissue factor; TT, thrombin time; TXA2, thromboxane A2, TXB2, thromboxane B2.
Author contributions
M.S.H. and T.M. developed the conception of the presented idea and developed the theoretical framework; T.M., M.A.S., S.M., and S.H. contributed to the final version of the manuscript. M.S.H. did the overall supervision and critic review for the manuscript. Authors have accepted responsibility for the entire content of this manuscript and approved its submission.